Creating Stretchable Thin Film Transistors from Inorganic Materials
28-09-2022 | By Liam Critchley
Transistors have become one of the most used electronic components in technology today, so there is always an interest in creating newer and smaller transistors that outperform their predecessors, allowing more efficient computing operations. However, it’s not just computers where there’s an interest in transistor innovation. The development of new types of electronic device, namely stretchable electronic devices, has prompted new developments in transistor technology that focuses more on them being able to conform to specific geometries and be stretchable rather than just looking at miniaturising alone.
As the interest in stretchable electronics grows, be it from electronic skins, wearable monitoring devices or soft robotics applications, the need for electronic components that can stretch with the device also grows. Many transistors are inorganic in nature, so they are typically less stretchy and flexible than components made of organic materials, but ways of incorporating inorganic materials into stretchable transistors are being sought.
Several pathways have already been trialled, but most of these only use the inorganic materials periodically, leading to challenges in terms of integration density (number of transistors per unit area) when built into larger electronic systems. A new approach has now been trialled by researchers where inorganic thin films are used to make an inorganic transistor that actually stretches.
Transistors for Stretchable Electronics
It’s no secret that transistors constructed from inorganic semiconductor materials are superior in performance and reliability compared to organic transistors. However, inorganic materials are typically much more rigid and brittle than organic materials meaning that organic transistors are much more stretchy.
The use of inorganic materials in stretchable transistors is not entirely unheard of; however, they have to primarily be placed within non-stretchable parts of the device so that they don’t undergo mechanical strain and deform at the interconnects. Because of this, there is typically a trade-off that needs to be made in terms of the integration density if the stretchability of the component is the main priority.
Overall, two main approaches are currently utilised to fabricate stretchable transistors. The first strategy is to use intrinsically stretchable organic materials to build the transistors and make the circuits stretchable. This approach has yielded some excellent results in terms of stretchability, as these devices can stretch up to 100% of the strain and maintain charge carrier mobility. However, even though they retain their mobility, the organic nature of these transistors means that their charge carrier mobility is still too low for some high-speed applications, limiting their application scope.
The second approach, which is the one that involves inorganic materials, is to build functional islands that are almost completely decoupled, with strain and stretchable wiring facilitating the entire deformation of the device and the functional islands being electronically connected via serpentine bridges. This approach has focused on higher device performance (because inorganic materials are used) but has also suffered from its own set of challenges.
In these approaches, the device performance is much higher than stretchable organic transistors. However, because the functional transistor elements are located on islands, the integration density tends to be low because the number of stretchable interconnects needs to be greater than the number of rigid islands to accommodate the high strain and mechanical deformations that are typically applied to the transistor. So, while these devices are functionable, the transistors are much bulkier than they should be for thin stretchable electronic devices, so an approach to creating thin film transistors with a high integration density and performance is being sought.
Creating Thin Film Inorganic Transistors
To overcome the trade-off between stretchability and performance, researchers have now developed an inorganic oxide thin film transistor where the devices are directly embedded into serpentine strings—rather than using the serpentine strings as bridges between rigid island regions. The thin film transistors on the serpentine host strings were then embedded into a flexible polymer support, in this case, polyimide (PI).
Fig. 1: Stretchable inorganic transistors with high integration density.
Because the transistors are embedded into the polyimide, they are not hidden from the deformation that is exerted onto the sheet, but because they are thin films and are inorganic in nature, they can endure the strain when flexed. This approach enables the transistors to endure strain up to 100% by themselves, so the integration density can be enhanced when they are directly embedded into polyimide sheets without sacrificing stretchability—because they flex as well, there is no need to form islands, and therefore more transistors can be integrated per area than when the ‘island’ method is used. This also means that the integrated transistor devices can be made smaller than the other inorganic island routes.
The direct fabrication of the thin film transistors into the polymeric host is seen as a favourable method compared to transfer printing separately made devices that have to be integrated—because it is a more straightforward process that allows for a greater number of transistors per area and can be done quicker. The process developed by the researchers generates a dual-gate architecture where the metal oxide transistors are integrated into the polyimide support at low processing temperatures (less than 300 °C).
Because the transistors are on serpentine strings within the polyimide, they can also bear some of the strain (unlike in other approaches), meaning that the stresses are not placed entirely on the polymer support, reducing the stress on the overall device, and indicating that the device can be stretched much more easily and for more extended periods.
In the device created, the stretchable inorganic transistors themselves were not only high performing (as expected for transistors of this type), but they also had a high degree of mechanical and electrical stability as well. In terms of the complete device, the researchers were able to integrate more than 30,000 transistors per cm2. The device’s electrical characteristics were preserved after being stretched to 100% strain. This is partly due to the stretchable nature of the transistors themselves and the polyimide support, showing that the device could be used for long periods compared to other stretchable inorganic transistor devices.
Because the fabrication approach is based on standard semiconductor and display fabrication techniques, it not only enables uniform device characteristics to be achieved but also offers a relatively simple approach for fabricating highly miniaturised stretchable components that require both high performance and reliability—something which many other stretchable inorganic transistors do not. While the research is still relatively new at this stage, it’s thought that the method could be adapted for large-scale integration manufacturing, where some potential applications include high-fidelity deformable sensors and expandable displays with a high resolution.
Reference:
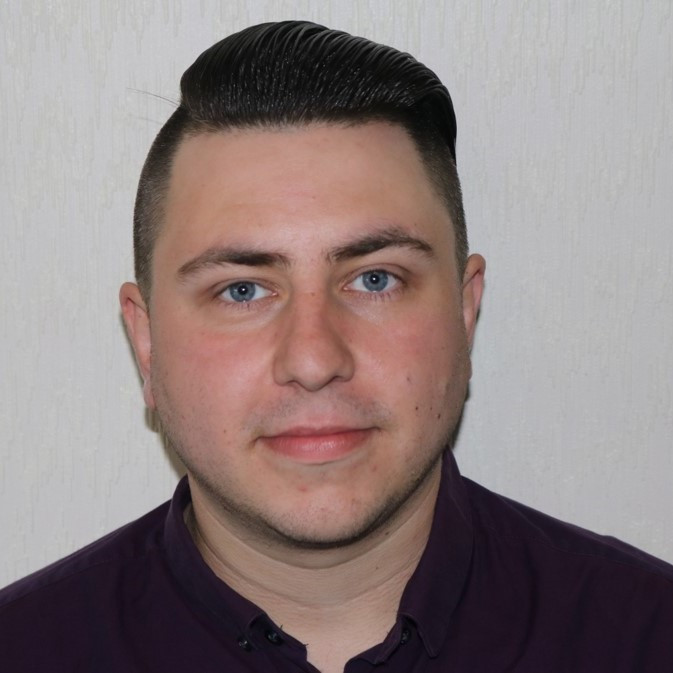