Solar Hydrogen Production Using Perovskites & Biomass
Environment | 01-11-2022 | By Liam Critchley
Solar hydrogen production is seen as one of the key technologies that could unlock a carbon-neutral, sustainable society, and it is often referred to as the ‘holy grail’ green technology when it comes to harvesting unlimited but intermittent solar energy. Solar hydrogen production is a relatively simple process and has undergone a lot of research over the last few decades, but despite the time and research investment, commercialising it on a wide scale for everyday use has been a challenge.
Solar hydrogen production requires a series of photo-electrochemical processes for it to work, but some of these steps—such as the water oxidation half-reaction—are very energy intensive. When coupled with the fact that many conventional inorganic photocatalysts have a relatively poor performance, there have been several big hurdles for solar hydrogen production that have prevented it from becoming a practical reality. Even with state-of-the-art catalysts, costs have still been high because the process still requires a lot of energy.
A lot of research is still going into making these systems more commercially feasible, and while we’re still a way off, new research is bringing about interesting developments that could be taken further in the future. One of the recent developments to come out has looked at using perovskite-based photocathodes and using lignocellulosic biomass as a source of electrons—as opposed to using water, to bring down the amount of energy required, as this is where the photocells are traditionally most energy-consuming.
Choosing Biomass Over Water
Water has been the medium of choice as an electron source in solar hydrogen production systems, but the energy required to initiate the oxidation half-reaction means that other materials could be a better source of electrons (from a commercial and cost perspective). Biomass is considered one of the most promising alternative electron sources for solar hydrogen systems because it is cheap, clean, carbon-neutral, and found in abundance. Biomass can also be oxidised at a lower bias, and there are potentially some extra economic benefits that could be realised from the oxidation by-products produced during the reaction.
However, there are still some issues with using biomass currently, including around global food ethics and economic feasibility when using processed chemicals derived from edible biomass. However, this does not apply to all forms of biomass, and lignocellulosic biomass is one type that can be explored more for solar hydrogen production—despite not being looked at much yet because it has a lower solubility and more complex molecular makeup that has traditionally resulted in lower yields and reduced selectivity.
The Issues with Photocatalysts
Photocatalysts are another key component of solar hydrogen production, but the low-level commercialisation of these technologies is in part due to the photocatalysts used (and available at an economically feasible price). Many photocatalysts used to date have a low performance. Conventional inorganic photocatalysts, such as TiO2, WO3 and BiVO4, are cheap to use and remain stable for long times, but they have large electronic bandgaps and poor optoelectronic properties.
These two properties mean that more energy needs to be put into the device to overcome the large bandgap, and it limits the use of solar flux—and often requires a large external bias that deteriorates the solar-to-hydrogen (STH) efficiency to well below the levels deemed feasible for commercialisation.
It’s thought that lead halide perovskite materials could offer a number of advantages over traditional photocatalysts. These perovskites have tuneable bandgaps and energy levels alongside a high absorption coefficient and excellent charge transport properties. These properties have already enabled several perovskite materials to become leading materials for the next generation of photovoltaic systems, and it’s thought they could offer similar advantages for solar hydrogen productions as well—but there have so far only been a few studies that have looked at perovskite-based photoelectrodes for solar hydrogen production, and none of the reports to date have been utilised alongside biomass as the electrons source.
Solving Some of the Key Challenges
Researchers have now tried to address some of the challenges with existing solar hydrogen production methods by looking at both low bandgap lead halide photocathodes as a panchromatic absorber and lignocellulosic biomass and source of electrons. The photo-electrochemical cell produced a record high photocurrent density of 19.8 mA cm-2 and also produced some valuable by-products—in the form of vanillin and acetovanillone—that could be used to add commercial value in indirect ways.
The high solar productivity, alongside a near-unity faradaic efficiency for hydrogen generation, was achieved by the researchers without any additional bias, nor were there any oxygen emissions causing trouble. Compared to water, the oxidative half-reaction for biomass had a much lower potential, and the perovskite photocathode was able to harvest the entire visible light spectrum as well.
Solid biomass was successful during this approach because the electrons were extracted using phosphomolybdic acid (PMA) as a catalyst and an electron/proton mediator. The PMA selectively depolymerises the lignin within the biomass, extracting and storing its electrons in the process while leaving the other components of lignocellulose (cellulose and hemicellulose) intact. This approach enables a range of different biomass forms to be used to produce solar hydrogen, including insoluble solid biomasses (such as lignin and lignocellulose) that are not typically usable in direct electrochemical oxidation reactions. The PMA reaction is also responsible for generating value-added chemical by-products.
The reduced PMA can also be reoxidised at a low potential, so PMA-enhanced biomass has the potential to replace the water in solar hydrogen production systems. The production system utilised by the researchers operated and remained stable for over 20 hours without degradation. The perovskite electrode was able to utilise the solar flux more effectively, opening the door to newer and different strategies for tackling the current issues with solar hydrogen production.
Reference:
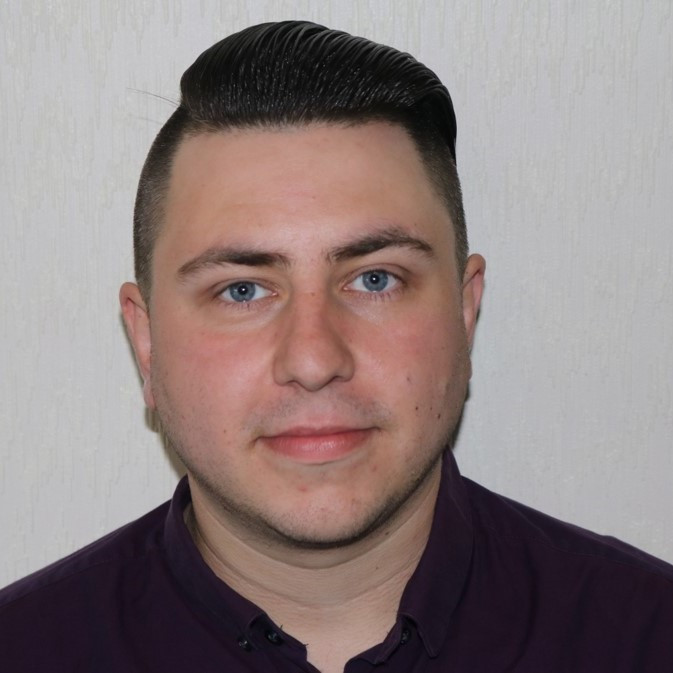