Understanding the Challenges of Rechargeable Metal Batteries
| 17-11-2021 | By Liam Critchley
In a market wholly dominated by the Li-ion battery, there is a lot of potential for rechargeable metal batteries—with lithium, sodium, calcium and magnesium metal batteries all being investigated. For this reason, rechargeable metal batteries are some of the most researched batteries at both an academic level and within the industry. This interest has arisen because many metal batteries can store more energy than rechargeable batteries that use carbon as the anodic material. However, while using metal as the anodic material has its energy benefits, several issues surrounding the interphase and interface chemistry of these metal electrodes need to be addressed before we see more extensive use of these metal batteries.
Some of the first batteries to make it into commercial technologies back in the 1980’s employed metal anodes. Metal anodes have since been phased out, favouring carbon-based anodic batteries such as the Li-ion due to safety concerns (as the older metal-electrode batteries used to catch fire easily). However, as the energy demands of modern-day society continue to grow, there has been a renewed interest in rechargeable metal batteries.
Despite the interest and further research towards their potential commercialisation, there are still many challenges, which is holding them back from seeing wider use. These are mostly centred around three areas—solid electrolyte interphase layers on the electrode, dendritic growth and electroplating, and issues with creating all-solid-state batteries where the electrolyte is also a solid-state material. We will look at it in more detail below.
Lack of Solid Electrolyte Interphase (SEI) Information
There are a number of chemical ion layers that can affect the performance of the rechargeable metal battery. For example, alkali and alkali earth metal compounds will naturally form a thin layer of their negatively charged constituents (carbonates, hydroxides, oxide species etc.) if certain environmental conditions are met (such as the presence of water of CO2). The formation of these layers can cause the electrolyte to become trapped and can potentially affect the performance before assembly has even begun (but these effects are thought to be minimal, if not negligible).
However, there is a more pressing issue than these naturally forming layers, and that is to do with the solid electrolyte interphase (SEI) layer typically found in rechargeable metal batteries. The SEI layer is essential for the battery as it can be used to provide excellent cationic conductivity. It helps with improving the long-term mechanical stability of the electrode. These SEI layers will typically form on the anode in the presence of salts, solvents, additives, and impurities in the liquid electrolyte. When alkali and alkali earth metals are used to create rechargeable metal batteries, these SEI layers can be several hundreds of nanometres thick and have many chemical constituents.
However, despite the importance of the SEI layer, there is limited experimental data into the characteristics of the SEI layer in metal batteries because the sensitivity of the analytical instruments used has not been great enough to provide distinct insights into the features and structure of these SEI layers. For example, it’s not known whether many of these SEI layers are amorphous or crystalline if they contain defects or not, or how the different chemical morphologies interact to provide beneficial (or detrimental) properties.
At this stage, some SEIs are unstable when certain metal/electrolyte combinations are used, and without knowing some of the specifics, it is almost impossible to tune the properties of the SEI layer (and the batteries in general). However, this does mean that with a bit more information regarding the SEI structure and dynamics in different metal batteries, there is still the potential to improve their performance and stability to levels that may be considered commercially feasible.
Dendritic Growth and Electrodeposition Challenges
Another issue is the level of electrodeposition on the electrodes. Metal electrode surfaces contain some dislocations, cracks and ridges where energetic ions can be deposited. In electrodeposition processes, metal ions from the electrolyte become reduced on the surface and settle into a zero valence (non-ionic) state. From here, the deposited ions form into a range of structures—most commonly dendrites—and this has a continual knock-on effect because the more ions deposited, the greater the surface area is for new ions to attach. The deposition of metal ions into structures like dendrites can have a range of consequences, including short-circuiting, increased cell resistance and the formation of electronically disconnected areas of the electrode. (commonly referred to as ‘dead lithium’ areas—but it does not apply to lithium-based batteries).
This is a challenge for rechargeable metal batteries because the electrodes are made of metals and not carbon-based materials such as graphite. Dendrites have been highlighted because they are the most common. Still, their formation can interfere with the SEI layer of a battery, and the kinking and buckling of dendrites can also create dead lithium/dead metal areas. This is a significant challenge for many metal batteries (as well as other batteries, such as lithium-sulphur). Not a lot has yet been done on a commercial scale to remedy the issues of dendrites. Still, it’s thought that very high currents could help smooth out dendrites by promoting a greater surface diffusion—and it is one of many different strategies being looked at to reduce electrodeposition and dendrite growth in rechargeable metal batteries.
Issues with Current All-Solid-State Batteries
There has been some work done to try and create all-solid-state batteries, which are batteries where the electrodes are not only solid-state materials, but the electrolyte is as well. The issues in these batteries are typically related to the electrolyte’s chemo-mechanical properties, as the electrolyte’s solid-state nature makes it prone to cracking. They can sometimes have poor electrical contacts, and any imperfections or defects in the solid-state lattice can cause dendrites to form.
One of the suggestions being trialled now is to use flat, atomically intact metal surfaces and alloys as electrode active materials and to use both heat treatment and high-pressure processes during cell preparation to improve the properties of the solid-state materials. There’s also been an interest in using sulphide-based electrolytes with more stable interfaces to try and address the stability issues of solid-state electrolytes. Beyond this, several computational models are being developed which could help to estimate the critical current levels where dendrites and voids form in the electrolyte materials.
Overall Outlook
Many different issues need to be addressed before rechargeable metal batteries become more of a commercially viable option. Beyond the problems stated above, there is a lack of consensus on the standard electrochemical cell set-up to investigate the different behaviours and characteristics of metal batteries. Some examples include flooded electrolyte conditions and anode-free systems, but neither of these are reminiscent of practical systems.
Once a standard testing set-up has been decided by the battery community, a lot more information about different aspects of the battery will be obtainable, which will help with tuning the optimal properties of the battery. As it stands, a lot more work is still required at a fundamental and industrial level to make rechargeable metal batteries a household name. However, there is still potential for these batteries in the future despite the many challenges currently being faced.
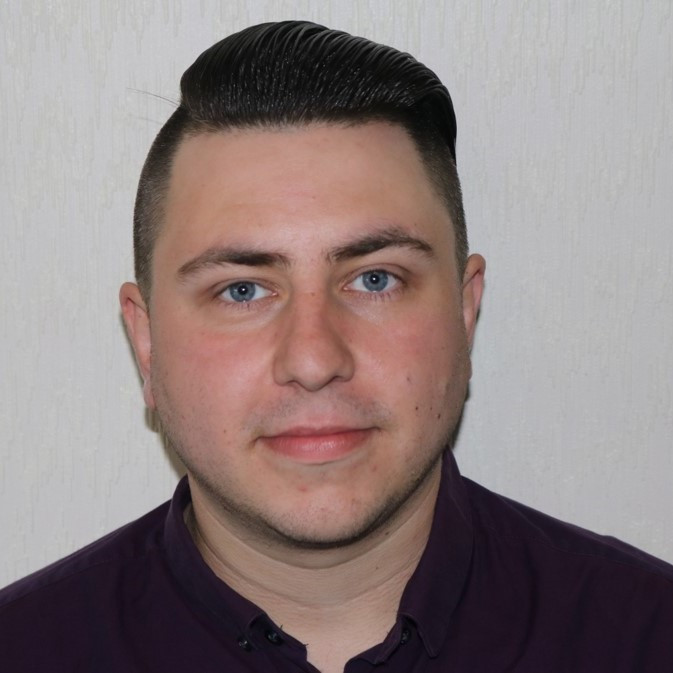