Smart NEMS for Implantable Medical Devices
07-07-2021 | By Liam Critchley
Very small and compact implantable medical devices (IMDs), that have wireless capabilities, are currently in demand for a range of healthcare monitoring applications. There is a particular interest in developing IMDs that can record neuronal activity by taking direct measurements of small neuron clusters to build up a picture of the widespread neuronal activity in a patient.
There is currently interest from both academia and the industry side for these kinds of technologies, as both fundamental research studies and clinical applications are interesting in the potential of such wireless monitoring devices. There are currently technologies available that can monitor the neuronal activity of a patient, but many of these devices are limited in where they can be used. There is a drive to build new IMDs that can expand the measurement scope and address the need for a distributed neural interfacing tool, and one of the recent devices to emerge has been built around nanoelectromechanical systems (NEMS).
What are NEMS?
A nanoelectromechanical system (NEMS) is similar to a microelectromechanical system (MEMS) in design, but they are nanoscale devices rather than micron-scale devices. As the name suggests, these devices integrated both electrical and mechanical functionalities into a single device at the nanoscale. The area of NEMS (and MEMS) is vast and the devices created are typically nanoelectronic devices and can be made out of a number of different materials.
The field is a growing area but is not yet as established as MEMS, but applications are emerging. Most of the current applications of NEMS are sensor applications—including chemical sensors, biosensors and accelerometers. Because NEMS are a system made up of different components that function as a single device, they utilise a range of nanotransistors, actuators, pumps and motors to perform sensing applications, and it’s thought that these systems could be implemented in IMDs to monitor neural activity.
Wireless Implantable Medical Devices (IMDs) and their Challenges
IMDs have been used for many years and perform a range of monitoring functions. However, to monitor neural activity, the IMDs need to be placed in and around the brain. Many of the IMDs available today are battery-powered (and use wires). Not only are wired implants uncomfortable (and very invasive) for the patient, but some are also too bulky to be placed in and around the brain, limiting their clinical use. This is why there has been an interest in creating wireless IMDs, and the wireless IMDs that are available today use either electromagnetic or ultrasonic waves to power the device.
However, even with many of the wireless IMDs, they are still very bulky. This is because the charging receivers in these devices need to be of a certain size so that they can deliver enough power to operate the IMD. If the receiver shrinks too much, it becomes difficult to operate the IMD as it puts too much pressure on the coils and piezo devices typically found within these types of IMDs. Depending on the application, anywhere from a few to several hundred microwatts is required, so conventional wireless IMDs are limited in their miniaturization capabilities.
Devices with miniaturized components have been created, but the wireless efficiencies are too low to be deemed safe for human use, but building these wireless IMDs around NEMS could offer a potential route for miniaturising wireless IMDs for neuronal activity monitoring while still being able to deliver enough power to render them safe for human use.
Creating IMDs with Smart NEMS
Researchers from Boston, USA, have previously undertaken work in this area and have created both a magnetoelectric (ME) NEMS acoustic wave resonator that acts as a single-band ME antennae for wireless communications in IMDs, as well as ME sensors (based on a contour mode resonator) that detect static low-frequency electromagnetic fields. As previous research has centred around 2 separate devices, the aim in their recent research looked to bring these together into a complete wireless sensing system that can be implanted in and around the brain to measure neuronal activity.
The smart NEMS antenna created in this research is only 250 × 174 µm2 in size and possess characteristics that are ideal for IMDs. The NEMS device created is a dual-band antenna that can switch between two acoustic frequencies of 2.51 GHz for wireless radio frequency harvesting (device charging) and 63.6 MHz that can perform neural recording based on magnetic field sensing. In short, the new NEMS device can perform both wireless charging and sensing functions, making them ideal for IMDs.
One of the main ways that this device has been possible is because of its transduction mechanism. Where other IMDs use coils that struggle to be miniaturised, the transduction mechanism in these NEMS devices are based around a magnetic-piezoelectric heterostructure that measures the H-components of electromagnetic waves. The sensing mechanism is based around the magnetic layer inducing an oscillating strain that generates a piezoelectric voltage output at the electromechanical resonance frequency—and this transduction mechanism is not privy to the same miniaturisation constraints that coils are.
Beyond miniaturisation, the compact antenna has a highly efficient power transfer efficiency, data communication in the GHz range, an ultra-sensitive magnetometer that can sense picoTesla level frequency fields and the ability to operate at both the 2.51 GHz and 63.6 MHz frequency bands. Moreover, the neural sensing mechanism is more localised than electrical sensing, leading to a better spatial resolution of neuronal activities, and the compact size of the NMES device can be used in both central and peripheral nervous tissue. Moreover, the devices can easily be coated with biocompatible polymeric coatings to keep them safe from biofouling once implanted and prevent them from being attacked by the body.
These recent devices have been able to solve the challenges surrounding simultaneous wireless charging and miniaturisation in IMDs that have made wireless IMDs unfavourable and unsafe for neuronal monitoring in humans. Now, that foundations have been laid into potential routes that can solve these challenges, the door is now open to see if these types of advanced nanotechnology systems are suitable for real-world clinical applications.
Reference:
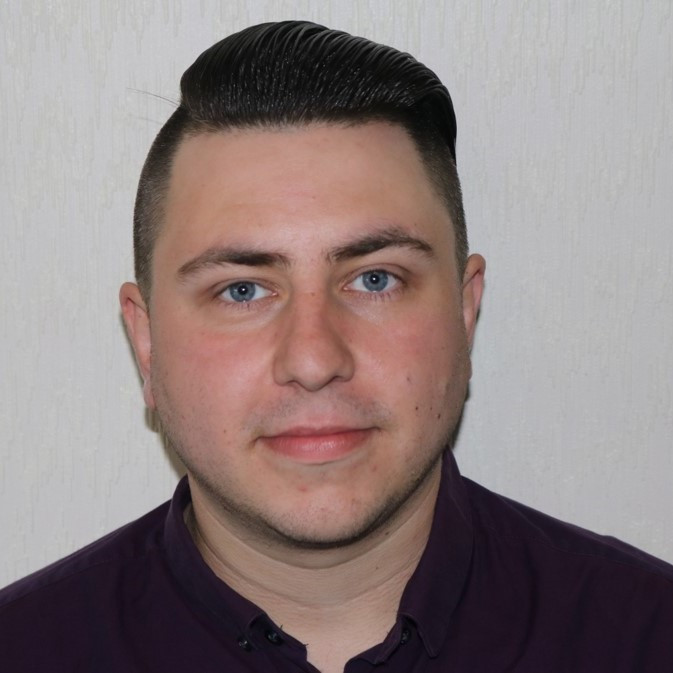