The Future of Bio-Informational Engineering
| 18-02-2021 | By Liam Critchley
Over the years, the interface of biology and informational systems has become more commonplace. Many systems are now being created that mimic the properties of biological systems for synthetic, informational gain. In some cases, it goes further than pure biomimicry. The direct integration of biological materials into information and data systems (such as DNA or RNA strands) has become commonplace.
While there has been an emergence of bio-inspired and bio-integrated information storage systems, another area of bio-informational engineering is concerned with the actual detection of data, rather than the storage and processing of the obtained data. In some cases, biological systems can be involved with both the detection of the data and the transfer and storage of the data. This is because the biological components within hybrid systems can act as sensor arrays that can translate non-traditional information into the digital world.
It’s safe to say that the applications of bio-engineered information systems are often different from traditional systems. Still, they also have an inherent ability to be integrated into synthetic systems because they behave similarly. Overall, pure biological systems tend to use nucleic acid biomolecules for storing information and metabolic processes to process energy.
While these processes can be harnessed for all-biological information devices, they can be applied to synthetic systems. For example, metabolic processes rely on redox reactions (i.e., reduction and oxidation reactions that involve moving electrons around like many human-made electronics), nerve cells transfer information using electrochemical action potentials making them similar to an ionic current, and an electrical current can be passed between cells and bacteria using proteins (specifically protein nanowires). Additionally, many biological systems can convert light into other forms of energy or output signals, as human-made systems can.
So, the basic, fundamental architecture is there for biological devices to be integrated with human-made systems. Because some biological molecules can store more information than synthetic chips and/or can obtain data that it otherwise difficult to obtain, the future of bio-informational engineering looks sets to advance further in the coming years. Naturally, many more challenges are present in such hybrid systems, but there is a lot of work going into the area.
Here, we look at how these hybrid architectures are being used in biosensing and optogenetic platforms (two of the most common fundamental areas) and future challenges. Beyond these two essential functions/components lies a range of applications that could integrate these hybrid bio-informational systems. These include general biosensing and information storage devices and communication pathways for the Internet of Biological Things (IoBT), satellite communications, and bioelectrical interfaces that can accurately interact, detect, and monitor biological environments within medical settings.
Biosensors
Biosensors are key areas where bio-information systems can be of use due to the difficulty of sensing and monitoring biological processes and environments (and the biocompatibility required to do so in more invasive detection approaches). There are now several biosensors which are made up of biological materials (for higher biocompatibility and binding affinity to the target of interest), and these can be coupled up to data transfer and storage networks (biological, synthetic, or hybrid) to provide an effective platform for sensing biological processes and specific biomolecules.
Some of the biosensors created in this way have used everything from various biomolecules to cells, DNA and RNA, genetically encoded biomaterials/cells and synthetic co-cultures as the sensing surface. In these biological biosensors, the sensing surface typically attaches to the biomolecule of interest. This molecular attachment then causes the sensing surface to undergo a conformational change, which invokes a different output response (electron transfer, fluorescence, gene expression etc.) that is detected and recorded.
With hybrid systems, there are always challenges marring the synthetic with the natural. Even though many biosensors are created to date that uses biological sensing surfaces, there are still many challenges before seeing them in a lot of real-world scenarios. The first challenge is a lack of target domains for every possible biomolecule that you might want to sense. Even though many of them can be functionalised/encoded to attach to almost all biomolecules, many do not exhibit a conformational change in the sensing surface when they bind, so no detectable output can be measured using this approach.
The second issue is that there is a lack of standardised biosensor architectures. A standardised and modular architecture would allow for routine integration of signal response, actuation, and output components. There has been a lot of progress in this area, but more work is still required as the current solutions are complex.
While both issues are present, work is being done to rectify them. Biologically based biosensors are critical for enabling biological signals to be converted into a digital output, so there is still a lot of potential for the future in this area, including the use of such biosensors in real-time monitoring of pollution and environmental contamination, agricultural and cellular production systems, and for tracking of health-related markers in bodily fluids and the gastrointestinal tract.
Optogenetics
Optogenetics is a biological field that uses wavelengths of light to control proteins, specifically neurons, within biological environments. As neurons are essentially the biological version of a communication channel, the ability to control these properties in a hybrid system has opened the door for optogenetics to be used in communication systems.
There are two main ways in optogenetic systems work, both of which are possible thanks to the ability to induce conformational changes in the presence of light. The first mechanism uses embedded enzymes to create ‘switch domains’ which changes in the presence of specific wavelengths of light. The second are modular systems that use proteins to induce and reverse signal outputs and control a range of processes it is attached to.
There is a range of potential areas that could benefit from optogenetic systems. One example is the use of optogenetic light emitters in smart culture bioreactors (using bacteria-based biosensors) to produce fine chemicals, pharmaceuticals, fuels and other materials/chemicals. Other examples of where optogenetic systems could be used include assisting sensing systems for the real-time monitoring of agricultural settings and control systems in biomedical applications. The latter could include real-time health monitoring using optogenetic wearable devices with bioelectric monitoring properties that can send the data to smartphones. Like biosensors, there are many possible opportunities at the interface of biological systems and data systems.
However, just like biosensors, there are also several challenges to overcome before they become widely used. One of the main challenges in this area is finding enough photoreceptors that can enable multiplexing operations to occur—and in turn, more complex functions to be performed. Other small challenges, such as the inability of light to penetrate high-density biological tissues/cell cultures, and the overexpression of proteins in some systems. However, there are ways that these smaller challenges can be overcome (e.g., specific engineering of the proteins and fine tuning of light exposure).
Conclusion
The very nature of biological-digital hybrid systems means that there will be inherent challenges coupling synthetic principles with biological mechanisms. Nevertheless, we see a lot of interest at all levels, from the sensing/data collection aspect to communication lines, and even in the transfer and storage of data itself. Biosensors and optogenetic platforms offer the most potential from a non-storage perspective.
While there are still a few challenges to overcome in these areas, there is a lot of potential for the future, including a range of applications and industry areas that could benefit from bio-informational systems. Time will only tell whether we see the adoption of bio-hybrid systems over all-synthetic systems. It will most likely depend on the ability to iron out the various challenges presenting the area currently.
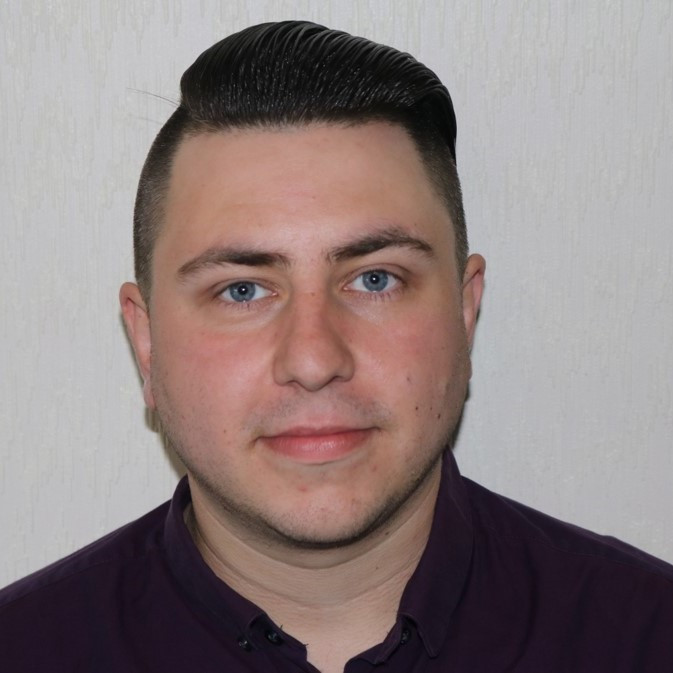