The 3D-Printed Sweat Collector Changing the Face of Wearable Sensors
08-06-2023 | By Robin Mitchell
Recently, researchers from the University of Hawaii demonstrated a new sweat collector developed using 3D printing technologies that could help pave the way to cheaper body-sensing solutions. Wearable biosensors are a rapidly evolving technology with the potential to revolutionize healthcare and wellness. These devices can noninvasively and continuously screen for biochemical markers in body fluids, providing valuable data for the prognosis, diagnosis, and management of diseases, as well as fitness monitoring1.
The research paper titled "Skin-interfaced microfluidic systems with spatially engineered 3D fluidics for sweat capture and analysis" was authored by Chung-Han Wu, Howin Jian Hing Ma, Paul Baessler, Roxanne Kate Balanay, and Tyler R. Ray. The paper was published in Science Advances on May 3, 2023.
In this article, we ask, what challenges do wearable sensors face, what did the researchers develop, and how could it help with other sensing technologies?
What challenges do wearable sensors face?
While still in their infancy, wearable medical devices possess an immense amount of potential to change the medical industry. The ability to gather medical data at all times not only allows for immediate responses to changes in health but can help power AI systems capable of providing a predictive medical diagnosis. But for all their advantages, trying to develop and deploy wearable medical devices presents numerous challenges, which is why they have struggled to become mainstream.
Accuracy
One of the primary challenges with wearable sensors is ensuring the accuracy and reliability of the collected data. Factors such as sensor placement, movement artefacts, and environmental conditions can affect the sensor’s performance and result in inaccurate readings. For example, if a heart rate monitor is not positioned correctly on the wrist, it may fail to capture accurate heart rate data. Additionally, sweat or moisture can interfere with the sensor’s functionality, leading to unreliable measurements.
Power Management
Another significant challenge lies in power management. Wearable sensors are typically battery-powered and need to operate for extended periods without frequent recharging. Hence, the need to balance power consumption with the desired functionality and data collection frequency. Low-power design strategies, such as optimising sensor sampling rates, utilising energy-efficient components, and implementing intelligent power management algorithms, are essential to extend the battery life and enhance the user experience.
Data Security and Privacy
With the increasing amount of personal data collected by wearable sensors, ensuring data security and privacy is of utmost importance as wearable sensors often transmit sensitive information, such as health records and biometric data, wirelessly to other devices or cloud storage. Thus, protecting this data from unauthorised access and data breaches and ensuring compliance with privacy regulations is a significant challenge.
User Acceptance and Comfort
For wearable sensors to be widely adopted, they need to be comfortable and seamlessly integrated into daily life. Bulky or uncomfortable designs can deter users from using them consistently, meaning that wearable sensors should be lightweight, flexible, and non-obtrusive to ensure user comfort and acceptance.
While wearable biosensors hold great promise, there are still significant challenges to overcome. These include the need for improved accuracy, reliability, and durability, as well as the need to address issues related to user comfort and device aesthetics2. Additionally, there are regulatory and privacy concerns that need to be addressed as these devices become more prevalent2
It's fascinating to see how these challenges are being addressed by researchers. The development of wearable sensors is a complex process that requires a delicate balance between technical functionality and user comfort. It's not just about creating a device that works; it's about creating a device that people will want to use regularly. The challenges are significant, but the potential benefits for healthcare are enormous.
Researchers demonstrate wearable 3D printed sweat collector
Researchers in North America are leading the development of innovative wearable platforms that can comfortably comply with the human body and efficiently sample fluids such as sweat, interstitial fluids, tear, and saliva for the electrochemical detection of biomarkers1. These advancements have led to pioneering developments in wearable electrochemical sensors, from minimally invasive continuous glucose monitors for chronic disease management to non-invasive sweat electrolyte sensors for dehydration monitoring in fitness applications1. Recognising the challenges faced with wearable medical sensors, researchers from the University of Hawaii demonstrated a newly developed sweat collector called the “Sweatainer” that is entirely 3D printed.
As off-putting as sweat can be, it can provide great insight into an individual’s health, as the chemical makeup of sweat is highly sensitive to changes in the body. For example, those who suffer from diabetes will often see elevated levels of sugar and volatile compounds in their sweat, going so far as to give sweet and fermented odours. At the same time, dehydration can be determined via a reduction in sweat production for any given activity, and it is also believed that sweat can help with determining conditions such as heart disease.
But trying to gather sweat for diagnostic purposes comes with some disadvantages. One major challenge is that trying to gather enough sweat for analysis is not an easy task. Pads can be placed in key areas, but while these do absorb moisture, they can readily dry out, eliminating a key component of sweat; water.
To get around this, the newly developed collector is able to take advantage of microfluid channels that slowly absorb sweat and then collect it into individual pods. From there, these sweat samples can be tested via specialist medical equipment to provide in-depth medical data. At the same time, it is possible for individual pods to contain specific reactive compounds that can provide test results via a change in colour. For example, it could be possible for one pod to be filled with an enzyme that reacts with glucose to change its colour.
Fig 1. Fully enclosed 3D-printed sweatainer. S Let's take a closer look at the 3D-printed 'Sweatainer', which is manufactured using a unique three-step print-pause-print process. (A) To understand its construction, imagine an exploded view of the Sweatainer, where you can clearly see the two distinct print processes. The first print is depicted in grey, laying the foundation, while the second print, shown in green, builds upon this base. (B) A photograph of one of the reservoirs after the second encapsulation print provides a tangible sense of the device's physicality. (C) The Sweatainer's performance is further demonstrated through a sequence of photographs that showcase its Capillary Burst Valve (CBV) functionality. These images distinctly illustrate the filling steps, labelled as Stage 1 through 3, providing a clear visual guide to the Sweatainer's operation.
The researchers used additive manufacturing (AM), or 3D printing, to create the "sweatainer." This method allowed them to produce structurally complex objects with true 3D architectures. The use of 3D printing in microfluidics is well-established for the rapid, cost-effective fabrication of high-resolution templates for soft lithography. The "sweatainer" consists of a 3D printed microfluidic network of enclosed channels and unsealed reservoirs, a reservoir capping layer of PDMS (poly(dimethylsiloxane)), and a gasket formed from ultrathin biomedical adhesive.
Fig 2. Evaporation measurement of sweat in 3D-printed microfluidic networks The process of measuring sweat evaporation within 3D-printed microfluidic networks is depicted through optical images of the devices utilised in these measurements. These images correspond to the highest (labelled as 'A', Device #2) and lowest (labelled as 'B', Device #3) evaporation rates recorded at three different time intervals: at the start (0 hours), after 2 hours, and after a full day (24 hours). The scale bars in these images represent 5 millimetres.
The researchers conducted their study by first designing the "Sweatainer" using computer-aided design (CAD) software. They then used a commercial digital light processing (DLP) 3D printer to fabricate the device. The "Sweatainer" was designed with a network of enclosed channels and unsealed reservoirs, which were created to slowly absorb and collect sweat.
To test the effectiveness of the "Sweatainer", the researchers conducted a series of experiments. They found that the device was able to collect multiple, independent sweat samples during a single collection period, a feature they termed "multidraw". This was a significant improvement over traditional single-use devices, which can only collect a single sweat sample at a time.
The researchers also found that the "Sweatainer" was able to maintain the integrity of the collected sweat samples, even under mechanical stress such as finger pressure or device removal. This is a crucial feature, as it ensures that the sweat samples remain pristine and suitable for analysis.
In terms of the potential applications of the "Sweatainer", the researchers suggested that it could be used in conjunction with wearable sensors to provide a more accurate and reliable method of monitoring health indicators. They also noted that the use of 3D printing technology makes the "Sweatainer" easy to manufacture and potentially accessible to anyone with access to a 3D printer.
The researchers introduced a novel mode of sweat collection, termed "multidraw," which overcomes the inherent limitations of single-use devices by enabling the collection of multiple, independent pristine sweat samples during a single collection period.
Fig 3. (A) A detailed rendering reveals the essential components of the Sweatainer system and its skin interface (port), including poly(dimethylsiloxane) or PDMS. (B) A photograph shows the Sweatainer affixed to the underside of an individual's forearm before sweat collection begins. (C) The design of the Sweatainer prevents unregulated fluid movement under mechanical stress, such as finger pressure or device removal. (D) An illustration of the Sweatainer emphasises key features of the device, including the inlet, capillary burst valves (CBVs; indicated by the blue and red dashed area), the collection reservoir, and ventilation holes to alleviate backpressure. (E) Three-dimensional (3D) renders of CBV designs made possible by 3D printing, with diverging angles of 90° (top) and 135° (bottom), are presented. (F) 3D printing facilitates the creation of device geometries in a true 3D space, as demonstrated by the computer-aided design (CAD) render (top) and a photograph of the actual device (bottom). The location of sweat is shown in blue. (G) A sequence of photographs illustrates the complete filling of a sweat collection reservoir. (Click to enlarge)
However, what makes the sweat collector of particular importance is the fact that it is made using a 3D print design, which not only simplifies its manufacture but could potentially make it available to anyone with access to a printer. According to the researchers, their collector was able to be manufactured using a commercial digital light processing printer (DLP), something which is readily becoming more affordable and available. The researchers noted that the fabrication of a fully enclosed microfluidic channel with feature sizes at the xy plane resolution limit of current DLP printers is dependent on several factors, including design aspects, print process parameters, and printer hardware. They also mentioned that the optical transparency of a 3D-printed microfluidic device depends on factors such as material selection, printer hardware, postprocessing, and surface roughness.
“3D printing enables an entirely new design mode for wearable sweat sensors by allowing us to create fluidic networks and features with unprecedented complexity. With the Sweatainer, we are utilizing 3D-printing to showcase the vast opportunities this approach enables for accessible, innovative and cost-effective prototyping of advanced wearable sweat devices.” - Tyler Ray, Department of Mechanical Engineering Assistant Professor.
The technical details of the 'Sweatainer' are impressive, and they highlight the potential of 3D printing technology in the field of wearable sensors. The ability to create complex structures with this technology could revolutionize the design of these devices. However, it's important to remember that the success of these devices will depend not only on their technical capabilities but also on their acceptance by users
The development of the 'Sweatainer' is a prime example of how innovative thinking and advanced technology can come together to create solutions to complex problems. The use of 3D printing technology in this context is particularly exciting, as it opens up new possibilities for the design and manufacture of wearable sensors. It's a reminder that sometimes, the key to overcoming challenges lies in looking at them from a completely new perspective.
How can this new device help with other sensing technologies?
While many other news outlets have been referring to the Sweatainer as a sensor, it is, in fact, only a collection device. Regardless of this fact, the sweat collector developed by the researchers could very well be crucial for future wearable sweat sensors that will likely struggle to work with tiny samples of sweat. The device developed by the researchers will provide such sensors with a larger sample size collected over a period of time. This helps to reduce the needed sensitivity of sensors, and the ability to store sweat without drying out also makes the samples collected an accurate representation of what is being secreted by sweat glands.
Of course, the next step for the researchers is to find a method for combining the newly developed Sweatainer with some portable electronic device capable of reading the collected samples. Overall, what the researchers have developed is encouraging for the field of wearable medical devices and could help transform lives in the years to come.
The future of wearable biosensors is promising, with potential applications spanning from remote and personalised healthcare to wellness, and we can expect to see devices that are not only more sophisticated but also more user-friendly. As technology continues to advance, we can expect to see more sophisticated devices capable of monitoring a wider range of health indicators1. However, it's important to note that the success of these devices will depend not only on technological advancements but also on user acceptance and regulatory approval2 and issues of data security and privacy are addressed.
The potential of wearable biosensors is vast, but it's important that this potential is realised in a way that is safe and respects the rights of users.
References:
-
Jihong Min, Juliane R Sempionatto, Hazhir Teymourian, Joseph Wang, Wei Gao. (2021). Wearable electrochemical biosensors in North America. Biosens Bioelectron. 2021 Jan 15;172:112750. doi: 10.1016/j.bios.2020.112750. Epub 2020 Oct 26. Link
-
M. Stoppa and A. Chiolerio. (2014). Wearable Electronics and Smart Textiles: A Critical Review. Sensors (Basel). 2014 Jul; 14(7): 11957–11992. doi: 10.3390/s140711957. Link
- Wu, C.-H., Ma, H. J. H., Baessler, P., Balanay, R. K., & Ray, T. R. (2023). Skin-interfaced microfluidic systems with spatially engineered 3D fluidics for sweat capture and analysis. Science Advances, 9(18). Retrieved from https://www.science.org/doi/10.1126/sciadv.adg4272#supplementary-materials
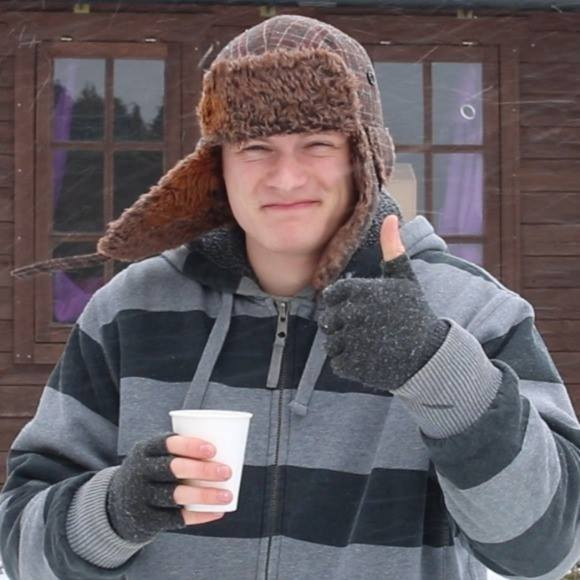