Reducing Auger Recombination in Perovskite LEDs
| 29-01-2021 | By Liam Critchley
Light-emitting diodes (LEDs) are common components in modern-day electronics. There are a number of different types of LEDs—both inorganic and organic—and a lot of research goes into LEDs as they are used in everyday items such as TVs. If you often buy/look up the latest TV, you will realise that the names are constantly changing based on the type of LED being used, and it is a very competitive area.
LEDs work by fluorescing light. In basic terms, when a current is passed through an LED, light is emitted from the device. LEDs are made up of semiconductor materials that have an inherent electronic band gap. This means that the charge carriers (negatively charged electrons and positively charged holes) are separated. This band gap is important for LEDs as it determines the wavelength of light emitted by the device, which is responsible for its colour.
Like many other areas of electronics, perovskites have been showing a lot of promise for LEDs. Scientists have started to go one step further using perovskite materials while harnessing the small size and quantum effects that nanosized structures have in emission-based electronics. This has led to the creation of quasi-2D perovskites being trialled for LEDs.
So far, quasi-2D perovskites that possess self-assembled quantum well structures have shown some promising results, including high emission efficiencies (quantum efficiencies) in both the near infra-red and blue light regions. There is the potential for these types of quantum-perovskite LEDs to be used in next-generation displays. However, current devices tend to suffer from a process known as Auger recombination. This is something that scientists are currently trying to mitigate so that 2D perovskite LEDs become a more commercially viable option.
The Auger Recombination Process
Auger recombination involves the transfer of energy to a third charge carrier (secondary electron), known as an Auger electron. When the electrons get elevated from a lower (ground) energy state to a higher (excited) energy state during any excitation process, it leaves a vacancy in one atom. An electron from the same atom can fill the vacancy left behind by the excited electron in some circumstances.
When the excited electrons return to the ground state, any excess energy is given off in the form of wavelengths of light. This is known as the hole-electron recombination process. However, when a third charge carrier has occupied the original vacancy site, most of the energy given off by the recombination process is absorbed by this charge carrier, and the Auger electron is ejected from the atom (and the atom returns to its normal state). So, instead of emitting a strong higher-energy wavelength of light, a lot of the energy that would be emitted is absorbed by the Auger electron, leading to a much dimmer light emission.
Auger Recombination in 2D Perovskite LEDs
Auger recombination in 2D perovskite LEDs is an issue because it means the devices suffer from an efficiency roll-off, so at low current densities, the efficiencies (and therefore the brightness) of the LEDs are greatly reduced compared to what they could theoretically be. In a world where there are already a number of bright LED devices, it is an issue that could limit the commercial potential of these devices if not remedied properly.
2D perovskites have very strongly bound excitons (excitons are the hole and the electron recombined) which are located within individual (discrete) quantum wells, as well as an efficient energy transfer mechanism, all of which can theoretically provide a very high photoluminescence potential (aka, a strong emission of light). However, the threshold for Auger recombination processes to occur within 2D perovskites is very low, meaning that they are more likely to suffer from Auger recombination losses more regularly—which is why there is a lot of potential, but not commercial realisation, yet.
There are a number of reasons why the Auger recombination threshold is low. One reason is that the carrier density at the recombination centres are orders of magnitude larger than 3D perovskite materials thanks to the high energy transfer efficiency of 2D perovskites. Auger recombination rate is proportional to the cube of recombination carrier density. So, in short, the better the energy transfer kinetics are at the recombination centre, the more likely the material is to experience Auger recombination issues.
Additionally, the strong exciton binding energy causes enhanced electron-hole interactions, which can also create some Auger recombination issues. In some cases, these enhanced interactions can cause the charge carriers to break free from their normal distribution. This non-uniform distribution increases the chances of more than one electron being near a single hole, leading to the second electron occupying the vacancy and becoming an Auger electron.
Trying to Overcome Auger Recombination Issues
A number of different approaches have been attempted over the years for reducing Auger recombination. This has ranged from engineering the perovskite's molecular structure to reducing the hole-electron wavefunction by altering the dielectric properties of the perovskite to the addition of new molecules that alter the overall properties and behaviour of the perovskite—particularly regarding the Auger recombination rate.
The addition of new molecules and structural engineering have been the most useful approaches for many materials that experience Auger recombination effects. Still, they have not been as widely implemented for 2D perovskite materials until recently.
This latest piece of research has focused on integrating high-polar organic cations, such as p-fluorophenethylammonium (p-FPEA+), into the perovskites structure to weaken the dielectric confinement around the recombination sites. This approach reduced the Auger recombination, but it also decreased the quantum photoluminescence efficiency, which is not ideal for LEDs. The organic cations occupied the defect areas and under-coordinated atoms of the perovskite that often contribute to the non-uniform electron distribution within 2D perovskites, lowering Auger's potential recombination.
To overcome the drop in efficiency, the team molecularly passivated the perovskite's surface (making it less active), which led to a drop in the Auger recombination—a lower amount of surface charge carriers lowered chance of Auger recombination—while keeping the efficiency high. The process also lowered the device's Joule heating effects, leading to a greater degree of stability.
In a world where LEDs are being used more and more, this kind of work helps to open new avenues of exploration commercially. Quantum LEDs (QLEDs) are already making their presence known in high-end smart TVs, so the ability to create more, and different, quantum LEDS in the form of 2D perovskite materials could help to further the field commercially.
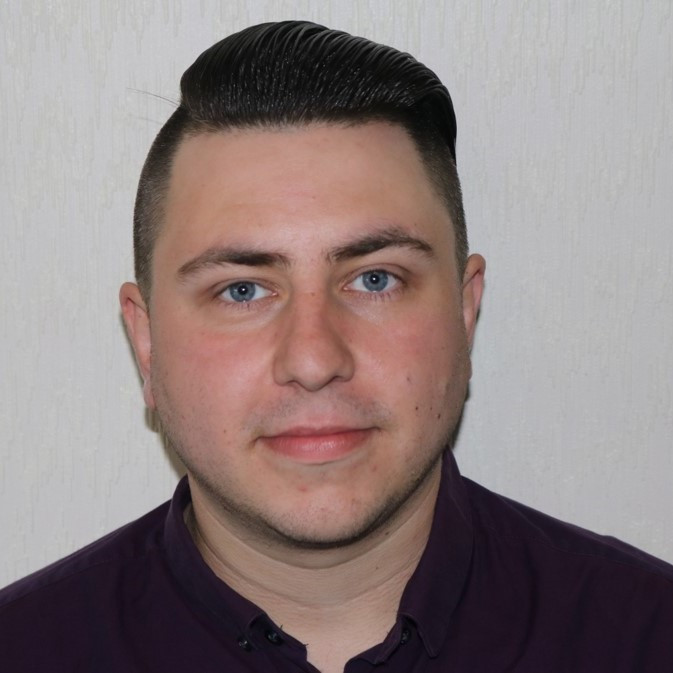