Wide Bandgap Semiconductors: The Key to Modern Military Technology
Insights | 21-05-2025 | By Gary Elinoff
Comparison of Silicon, GaN, and Sic Semiconductors. Image source: Navitas
Key Takeaways:
- Wide Bandgap Semiconductors handle more power at higher frequencies than traditional Silicon(Si) can
- They are also resistant to radar and do a better job of dealing with heat
- Designers must be aware of the difference between Switching Frequency and Operating frequency
Introduction
Western nations are ramping up their defense spending, and that doesn’t mean following Russia into an atavistic competition to see who can build more armaments suitable for a replay of WWI. No, the smart money is on smart military tech. High on the list are new, more powerful radars, jam resistant high frequency communications, missiles with greater range and accuracy and electronic countermeasures.
What it all adds up to is the need to operate at gigahertz-level frequencies and to do it with greater power than ever before. The new equipment may need to operate in space because even now, space is a theater of military competition and will be even more so in the future. Whether deployed in space, on an aircraft or on the ground, the need for a lighter, smaller kit that is increasingly rugged is paramount.
A large part of these issues can be addressed through the use of semiconductors based on SiC (Silicon Carbide) and GaN (Gallium Nitride). Known collectively as Wide Bandgap Semiconductors, they offer unique advantages over the classical Silicon (Si) based devices that they will largely replace.
What are Wide Bandgap Semiconductors?
Wide bandgap semiconductors (WBG) are based on materials with a wide gap between their conduction and valence bands. This is the source of the ability to handle more power for devices of the same size as gallium arsenide or silicon semiconductors. And compared to old-school Silicon, WBGs can operate at higher frequencies. They can serve to replace the travelling wave tubes that are employed as the power output stage for applications such as radar. This, again, saves weight and space and also improves mechanical reliability.
WBGs can not only operate at higher temperatures, but they also do a better job of transmitting the heat they generate, easing the challenge of dealing with thermal management. These are important factors for any type of electronic device, and perhaps even more so for military applications.
The Earth’s atmosphere and its magnetic field protect the planet’s surface from the high levels of radiation ever present in space. The military’s satellites, missiles and space stations no longer enjoy this shield, and the semiconductors that are their heart are quite vulnerable. However, when compared to classical silicon-based semiconductors, GaN and SiC devices offer different levels of protection against these hazards in an array of circumstances. This will translate into less need for mechanical shielding, saving still more weight and freeing up more area.
Hey, Wait a Minute – about those Frequencies!
Those of you from the power supply community must be scratching your heads, so let me clear up some possible confusion about WBG operating speed!
It’s well known that the ultra-fast 10 MHz switching range of GaN transistors makes them key components in voltage converters – but hold on. If GaN transistors can switch “only” in the 10 MHz range, what use are they in radar systems that operate at frequencies well in excess of a gigahertz? The answer lies in the difference between Switching Frequency and Operating Frequency.
- Switching Frequency is what we’re used to when we discuss things like voltage converters or EV traction converters. In these applications, Hard Switching takes place – the transistors turn completely ON and completely OFF, creating pulsating DC. For an explanation of this fascinating process, see "SiC and GaN Power Semiconductors Boost EV Driving Ranges.”
- Operating Frequency involves employing GaN Transistors in their small signal, linear modes. Here, they don’t turn completely on or off, but rather operate in an analog mode at much, much greater speeds, where they can be employed to amplify the GHz range sinusoidal signals that are the essence of radar.
It’s interesting to note that GaN’s switching speed is somewhat faster than SiC’s, but SiC devices can handle more power.
GaN and SiC Resistance to Radiation
The wide bandgaps that characterize SiC and GaN means that it takes more radiation energy to cause deleterious effects, as compared to Silicon. GaN might be stated to have more overall overall resistance, but the advantage isn’t cut and dry. Designers must consider the types of radiation expected, and over what time period the device is expected to operate.
GaN
As described by EPC[1], “Unlike silicon-based technology, where special processing is required to protect the semiconductor against the total ionizing dose (TID) of the effects of radiation, the physical properties and construction of gallium nitride (GaN) devices make it relatively immune to the damage caused by such radiation in space.”
Inside an Enhancement Mode (normally “off”) GaN. Image source: EPC Space
While GaN is inherently resistant to Gamma and Neutron radiation, Single Event Effects (SEE) in the form of massive heavy ion bombardments can be a problem. However, “Using special processing and design, eGaN devices can be made as immune to SEE as they are inherently immune to TID.”
SiC
SiC’s strong lattice structure protects it against energetic particles significantly. It is also resistant to the accumulative effects of radiation (TID) and massive Single Event Effects (SEE) when compared to Silicon.
GaN for Advanced Radar
The US Army has begun production of its Lower Tier Air and Missile Defense Sensor (LTAMDS). It is intended as a probable replacement for the radar system at the heart of the famous Patriot missile defense system.
The Lower Tier Air and Missile Defense Sensor is the next-generation air and missile defense radar for the US Army. Image source: RTX (Raytheon)
This radar, made possible by advanced GaN power semiconductors, is an example of an ASEA (Active Electronically Scanned Array) system. Unlike classical radars, they don’t sport massive, curved antennas which must be mechanically rotated through 360° to scan the sky. Classified as phased array antenna systems, they field large numbers of small electronic RF transmitters located on a flat plane. By varying the relative phase of each transmitter with respect to its peers, the resulting beam can be directed to any location through constructive and destructive interference.
A major advantage of the new LTAMDS is that it sports not one, but three planes of RF transmitters. Mounted at angle to each other, this new system can scan all 360° of sky at the same time.
There are many advantages to phased array systems.
- The direction of the beam, the sky space where targets are suspected and sought, can be changed almost instantly.
- They can form multiple beams simultaneously, tracking multiple targets at the same time.
- They can change transmitting frequencies often (frequency hopping), making it more difficult for enemies to pinpoint system location.
- There is no large, troublesome rotating mechanical antenna that causes reliability issues.
Jam-Resistant, High-Frequency Communication
Jam-resistant communications are vital to soldiers operating in an area where the opponent is employing electronic counter measures. Part of the solution is the ability of the combat radio to transmit a high power, high frequency RF signal to overpower the opponent’s jamming attempts.
This would be hard to achieve with traditional, silicon-based systems, due Silicon’s inherent limitations. Generating a powerful enough signal within the confines of a system small enough to be man-portable would be fraught with difficulty.
The solution, again, is WBGs. Weight is reduced due to the greater efficiency of WGBs in handling heat, thus reducing the need for heat sinks. Higher power density reduces space needs, and greater overall power efficiency means a smaller, lighter battery.
And finally, GaN can readily support the Ku-band and X-band frequencies common in military communications.
Modern Missile Systems
SiC means higher power capabilities, and missiles are high-power systems. They feature heavy-duty voltage converters and power units that must flawlessly control the missile’s steering mechanisms and actuators. SiC can also handle the weapon’s high-temperature environment, up to 600℃, where Silicon would fail.
Highly robust SiC semiconductors can handle the extreme demands of the missile’s take-off phase and are actually able to survive in the area of the nose cone.
Wrapping Up
WBGs, such as SiC and GaN have greater speed and power capabilities than classical silicon-based semiconductors. They also do a better job of handling heat, and they pack far greater power for a given area. And, important to space-based applications, they provide significantly better resistance to radiation.
Designers must realize that there is more than a two order of magnitude difference between switching speed and operating speed. This dichotomy doesn’t get the attention it deserves, and may be a source of confusion.
Thanks to WGBs, Western missiles will travel farther with greater accuracy, Radars will be more potent and versatile and will be resistant to enemy detection.
Because of the factors outlined, front-line troops will be empowered with jam-resistant, man-portable radios.
Challenges and Opportunities
Semiconductor manufacturers worldwide are striving to reduce SiC production costs and to increase manufacturability through larger wafer sizes. And SiCs and GaNs will not be the last word in WBGs.
Raytheon, a part of RTX, has been awarded a contract from DARPA to develop foundational ultra-wide bandgap semiconductors or UWBGS. These new devices will be based on diamond and aluminum nitride technology that will provide even greater power delivery and thermal management for electronic applications.
As per Raytheon[2], “The unique material properties of UWBGS offer several advantages over traditional semiconductor technologies, enabling highly compact, ultra-high power radio frequency switches, limiters, and power amplifiers. Their high thermal conductivity also allows the ability to operate at higher temperatures and in more extreme environments.”
References
- What is Radiation-Hardened Gallium Nitride? EPC Space
- RTX to develop ultra-wide bandgap semiconductors for DARPA. RTX (Raytheon)
Glossary of Terms
- TiD (Total Ionizing Dose). This is the amount of damaging radiation absorbed by materials such as SiC or GaN over an operational lifetime.
- ASEA (Active Electronically Scanned Array). A type of radar employing multiple transmitters to generate a beam pointing in any direction through constructive and destructive interference.
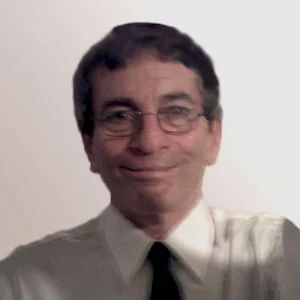