Paralysed Man Walks with 'Digital Bridge' Reconnecting Brain & Spine
In The News | 05-06-2023 | By Robin Mitchell
Recently, researchers have demonstrated a revolutionary technology that helps those with paralysis to regain motor capabilities, with one paralysed man having restored his ability to walk. Drawing from my experience as an electronic engineer and my understanding of the challenges involved in developing medical electronic devices, I will delve into the intricacies of this revolutionary technology. What challenges does neuron-electronic communication face, what did the researchers develop, and how could this technology revolutionise the medical industry?
What challenges does neuron-electronic communication face?
Advancements in medical science have transformed our lives as we know it; MRI and CAT scanners provide detailed internal models of the human body, which eliminate the need for invasive explorative surgery, numerous drugs that can cure even the most challenging diseases, and even genetic engineering that can reverse the effects of DNA defects that were formed during the first spark of life.
However, there are still many aspects of human health that remain challenging, if not impossible, to cure. Dementia is a significant problem affecting older generations, currently without a cure. While cancer continues to be an unlucky lottery that doesn’t always come with an easy cure.
But one medical condition that continues to allude researchers is paralysis, or specifically, paralysis due to neuron damage. Unlike the vast majority of cells in the human body, neurons are not so easily repaired when damaged, with nerve damage often being irreversible. With physiotherapy, is it possible to regain sensation and control after nerve damage, but this often requires for some neural a pathway to be intact as well as retraining of neural pathways. Thus, those who succumb to horrendous injuries that damage nerves need to essentially relearn how to do tasks such as walking and lifting.
Extreme nerve damage can make repairs impossible, and this can lead to permanent paralysis. It is in these cases that researchers often find themselves scratching their heads trying to come up with methods of restoring neural pathways.
Neurons are complex, and connecting them with a wire is not sufficient to restore neural pathways. Everything from electro potentials between axions to signal processing are required, and a single nerve bundle can contain thousands of potential connections. Grafts can be taken from different areas of the body, but they are not as simple as Lego pieces that can be stuck in the way between two different nerves.
Some researchers have been able to demonstrate basic systems utilising specialised electrodes and computational devices, but these typically provide minimal sensation or are faced with impracticality issues. For example, such devices need to graft small electrodes directly to nerve endings which introduce challenges with biocompatibility. Another example challenge is understanding the signals coming from nerves and how that data should be processed. Using traditional methods of noting down every signal pattern and matching what that does generally doesn’t work, as nerves can have many thousands of different signals and can change over time.
Researchers create a digital bridge that restores movement in a paralysed man
In a groundbreaking development in the medical field, researchers from Switzerland recently demonstrated a new digital bridge that was able to restore motion to a paralysed man, even going as far as to repair neuron damage. This breakthrough has been led by Grégoire Courtine, a neuroscientist at the Swiss Federal Institute of Technology in Lausanne. Courtine's previous work has demonstrated that technology stimulating the lower spine with electrical pulses can help people with spinal-cord injuries to walk again. This new device builds upon that foundation.
The research paper, titled "Walking naturally after spinal cord injury using a brain–spine interface," was authored by a team of researchers led by Grégoire Courtine, a neuroscientist at the Swiss Federal Institute of Technology in Lausanne. Courtine's previous work has demonstrated that technology stimulating the lower spine with electrical pulses can help people with spinal-cord injuries to walk again. This new research builds upon that foundation, demonstrating a breakthrough in the field of neurorehabilitation.
The new device forms a digital bridge between the brain and the paralysed area, acting as a transceiver for neurons on both sides. The first device is implanted above the region of the brain where motor controls are located, and the second device is implanted over the areas of the spinal cord that is responsible for the paralysed area.
"We previously showed that epidural electrical stimulation targeting the individual dorsal root entry zones of the lumbosacral spinal cord enables the modulation of specific leg motor pools. These stimulation sequences restored standing and basic walking in people with paralysis due to a spinal cord injury."
Figure 1: The design, technology, and implantation of the Brain-Spine Interface (BSI). 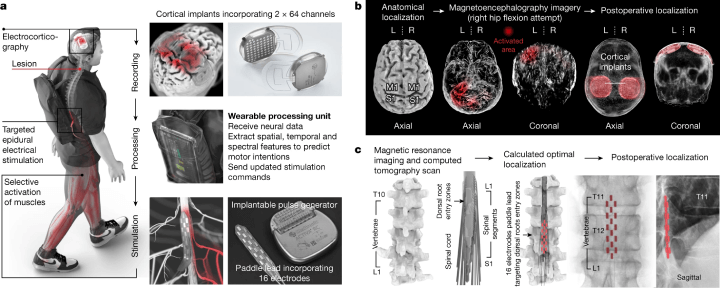
The digital bridge comprises two key components: (a) a pair of cortical implants, each with 64 electrodes, positioned over the sensorimotor cortex to gather ECoG signals. These signals are processed by a unit that interprets motor intentions and translates them into epidural electrical stimulation programs. These programs target the dorsal root entry zones of the lumbosacral spinal cord, with stimulations delivered via an implantable pulse generator connected to a 16-electrode paddle lead. (b) The process involves meticulous pre-operative planning for the placement of the cortical implants, which is confirmed post-operatively. (c) A personalised computational model is utilised to predict the optimal placement of the paddle lead to target the dorsal root entry zones associated with lower limb muscles, a prediction that is subsequently confirmed post-operatively. (click to enlarge)
The digital bridge works by integrating two fully implanted systems that enable the recording of cortical activity and stimulation of the lumbosacral spinal cord wirelessly and in real-time. This setup allows for the monitoring of electrocorticographic (ECoG) signals from the sensorimotor cortex. The decoded motor intentions are then converted into stimulation commands that are transferred to tailored software running on the same processing unit. This integrated chain of hardware and software establishes a wireless digital bridge between the brain and the spinal cord, converting cortical activity into the analogue modulation of epidural electrical stimulation programs to tune lower limb muscle activation.
Once implanted, patients attempt to perform specific actions, such as moving their hip or lifting their leg, and the signals from the bridge are recorded. From there, artificial intelligence is able to learn from the patterns and start to identify which signals refer to what action and what muscles need activating. The calibration of the Brain-Spine Interface (BSI) required two independent procedures to select the features of ECoG recordings that discriminate the intention to move and to configure stimulation programs that modulate specific ensembles of lower limb muscles. The first procedure consisted of extracting the spatial, spectral and temporal features of ECoG signals that were linked to the intention to mobilize each joint of both lower limbs. The second procedure consisted of configuring stimulation programs.
In the case of one 40-year-old Dutchman who had been left paralysed after a bike accident, he was able to regain some motor capabilities within 5 minutes after two days from the surgery. With a combination of routine and training, the system eventually provided the man with the freedom to move outside of a wheelchair, walk up and down stairs, and even be left unsupervised at home with the system operating.
"The BSI enabled continuous, intuitive and robust control of walking. When the BSI was turned off, the participant instantly lost the ability to perform any step, despite detected attempts to walk from the modulation of cortical activity. Walking resumed as soon as the BSI was turned back on."
The device's revolutionary aspect lies in its ability to stimulate damaged nerves, promoting growth. This is arguably one of the biggest challenges when suffering from paralysis, and why physiotherapy is so important; nerves that undergo stimulation are better at repairing themselves. As such, after one year of using the implant, the patient was able to turn it off entirely while still maintaining motor capabilities, providing clear evidence that the nerves had started to fix themselves.
"The participant reported that the BSI enabled a natural control over his movements during walking."
The stability of the BSI was assessed by quantifying the stability of cortical signals and decoders over time and the need to adjust stimulation programs. After a transitory one-month period, during which cortical signals exhibited modest changes in the spectral content of the different frequency bands, ECoG signals remained stable over the following months. This stability enabled robust performance.
The patient involved in this study, Gert-Jan Oskam, experienced significant improvements in his mobility. After around 40 rehabilitation sessions using the brain-spine interface, Oskam had regained the ability to voluntarily move his legs and feet. This type of voluntary movement was not possible after spinal stimulation alone, suggesting that the training sessions with the new device prompted a further recovery in nerve cells that were not completely severed during his injury.
How could this technology revolutionise medical care?
There is no doubt that what the researchers have developed is nothing short of a major breakthrough and could see many with paralysis given effective treatment to restore motion. Of course, the system has only been tested on one patient, but considering that the results from this experiment have been overwhelming, it won’t be long before the researchers look to new test subjects to confirm their results.
But restoring motion in those with paralysis from accidents isn’t the only potential application for the new digital bridge. It is also believed that it could be used in those who have suffered from a stroke, where brain damage prevents signals from reaching one side of the body and could aid in restoring motor capabilities, facial control and even improve speech.
The research suggests that the brain-spine interface (BSI) could have broad implications for treating various types of paralysis and other neurological conditions. The BSI works by converting cortical activity into the analogue modulation of epidural electrical stimulation programs to tune lower limb muscle activation. This could potentially be adapted to treat different types of paralysis, depending on the specific muscles and neural pathways affected. Furthermore, the research suggests that the BSI could potentially be used to treat conditions where brain damage prevents signals from reaching one side of the body, such as in stroke patients. This could aid in restoring motor capabilities, facial control and even improve speech.
For now, we will have to wait for more trials to be conducted and more data gathered before we can say for sure if this digital bridge will revolutionise health care. However, it seems that the results are, so far, overwhelmingly positive. The clinical study was designed to investigate whether neurorehabilitation supported by the BSI further improves neurological recovery. Before enrolling in STIMO-BSI, the participant had completed the clinical trial STIMO, which enabled him to regain volitional control over previously paralysed muscles and to improve his standing and walking functions. However, after three years of regular training with stimulation only, he had reached a plateau of recovery.
References:
-
Abbott, A. (2023). Paralysed people are walking, thanks to a revolutionary spinal implant. Nature. Retrieved from https://www.nature.com/articles/d41586-023-01728-0
-
Capogrosso, M., Milekovic, T., Borton, D., Wagner, F., Moraud, E. M., Mignardot, J. B., ... & Courtine, G. (2023). Walking naturally after spinal cord injury using a brain–spine interface. Nature. Retrieved from https://www.nature.com/articles/s41586-023-06094-5
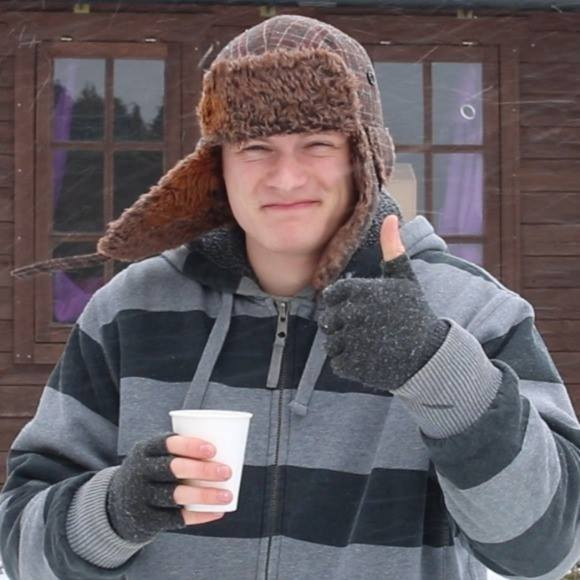