The Next-Gen Transistor: University of Michigan's Breakthrough in Ferroelectric Semiconductors
Semiconductors | 30-03-2023 | By Liam Critchley
Professor Zetian Mi's research group demonstrated the feasibility of a reconfigurable ScAlN/AlGaN/GaN ferroelectric HEMT transistor, which holds significant promise for the advancement of communication and computing systems in the future.
Graduate student Minming He (left) and Dr Ding Wang are collaborating on the development and fabrication of ferroelectric HEMTs utilising a novel nitride material, ScAlN. Recent research has identified ScAlN as a promising high-k and ferroelectric gate dielectric, capable of introducing new functionalities and enhancing device performance. Photo credit: Marcin Szczepanski, Lead Multimedia Storyteller, Michigan Engineering
Researchers at the University of Michigan have been doing extensive research recently into ferroelectric semiconductor devices. It was only last month [February] when the team, Wang et al., developed a ferroelectric semiconductor platform for use in AI and IoT computing systems, and the team are already back again with their latest offering―a ferroelectric semiconducting high electron mobility transistor (HEMT).
The Emergence of Ferroelectric Semiconductors
Over the past few decades, silicon has been the dominant material in the semiconductor industry, enabling the development of a wide range of electronic devices. However, as we continue to push the limits of device miniaturisation and performance, the limitations of silicon-based transistors have become more apparent. This has led researchers and industry experts to explore alternative materials and technologies that could potentially outperform silicon and provide new functionalities.
Ferroelectric semiconductors have emerged as a promising candidate in this search for new materials. They possess unique properties, such as the ability to sustain and switch electrical polarization, which can be harnessed to create more versatile, energy-efficient, and compact electronic devices. Several research groups and companies worldwide have been working on the development of ferroelectric semiconductor devices, aiming to overcome challenges related to material synthesis, integration, and scaling.
Notable advancements in the field include the introduction of hafnium oxide-based ferroelectric materials, which have shown potential in non-volatile memory applications, and the ongoing exploration of complex oxide materials for a variety of electronic and spintronic devices. The work conducted by the University of Michigan researchers on ferroelectric semiconductor HEMTs, as detailed in this article, represents another significant step forward in the pursuit of smaller, more efficient, and reconfigurable electronic devices.
As the demand for high-performance, energy-efficient, and miniaturised electronic devices continues to grow, so too does the need for innovative materials and design approaches. The development of ferroelectric semiconductors and their integration into various applications, such as AI and IoT computing systems, amplifiers, non-volatile memory devices, reconfigurable power devices, negative capacitance transistors, and radio frequency devices, showcases the potential of this emerging technology.
While many of these advancements are still in the research phase, the commercial potential of ferroelectric semiconductors is becoming increasingly apparent. The University of Michigan researchers' decision to apply for patent protection is a testament to their confidence in the technology's future applications. As more breakthroughs are made, and new devices based on ferroelectric semiconductor materials are developed, we can expect to see a growing impact on the electronics industry and a broader range of applications that could benefit from these advanced materials.
The Choice to Use Ferroelectric Semiconductors
The researchers chose to use ferroelectric semiconductor materials over conventional silicon-based architectures because they can easily sustain an electrical polarization. The ability to sustain this polarization means that the polarization can switch the ends that are positive and negative. This phenomenon offers a more flexible design architecture for transistor-based devices because the transistor can change how it behaves to perform different functions.
The addition of a ferroelectric domain into a HEMT also makes this switching capability much quicker and more efficient. A quicker switching speed between polarization states offers a way to create devices that have a lower power consumption, a higher gain, and an overall greater level of efficiency. Another benefit of using ferroelectric materials is that it enables the HEMT to be reconfigurable.
By building a reconfigurable HEMT device, it allows the device to perform the same function as several devices. This is why the researchers have previously investigated these ferroelectrics for small-scale computing applications, as the ability to create multi-functional devices means fewer components need to be integrated onto a chip, reducing its size.
The focus in the most recent research has been on utilising the ferroelectric materials in an amplifier―where one amplifier can perform the same function as several other amplifiers―and once again, size considerations were a key part of the driver to use the materials as multi-functional amplifiers offered a way of reducing the required circuit area and lowered the energy consumption of the device.
Fabrication of the New HEMT Device
Published in the journal Applied Physics Letters, Wang et al. have used molecular beam epitaxy to create high electron mobility transistors (HEMTs). The ferroelectric semiconductor is a material with the chemical formula ScAlN, which is an aluminium nitride material that has been spiked with scandium, and it has already shown high-k and ferroelectric gate dielectric properties to boost performance and offer new functionalities into a device. This material is also the first nitride-based ferroelectric semiconductor material and is compatible with gallium nitride (GaN) semiconductor materials.
GaN is another material that has gained a lot of popularity in recent years and has been one of the materials that have been proffered to replace silicon in electronic devices due to its high efficiency and low cost. Its compatibility with ScAlN provided the researchers with an opportunity to create heterostructures using the two materials. The heterostructure created by the researchers was a ScAlN/AlGaN/GaN-based ferroelectric heterostructure.
While a conventional transistor is a basic electronic switch within a circuit, the HEMTs developed by the researchers have many more functions because they can increase the gain (signal), provide high switching speeds, and have a lower noise than conventional transistors. These properties, especially around the increased gain potential, have opened the door to these HEMT devices being utilised as amplifier devices that could be used for sending high-speed signals to cell towers and Wi-Fi routers. Regarding its performance, the HEMTs showed a wide threshold voltage tuning range of 3.8 V and a large ON/OFF ratio of 3 × 107, showing that the device has fast switching capabilities.
The researchers have also stated the research undertaken in the latest study provided some insights into the ferroelectric polarization coupling and threshold tuning processes in ferroelectric nitride heterostructures and opened some new doors to creating multi-functional devices that could help to shrink down a range of electronic devices.
Conclusion
While the focus here has been on amplifiers, there is the potential to utilise these ferroelectric nitride heterostructure architectures in a range of devices, including non-volatile memory devices, reconfigurable power devices, negative capacitance transistors and radio frequency devices. The realization of this novel transistor also opens the possibility of integrating multi-functional devices into electronic systems, where the devices can perform the function of multiple components― such as reconfigurable transistors, filters, and resonators―all on a single platform. While the research is still at an academic level, the researchers are confident enough in the commercial potential of these devices that they have applied for patent protection, so it will be interesting to see if these multi-functional systems make it beyond the academic lab.
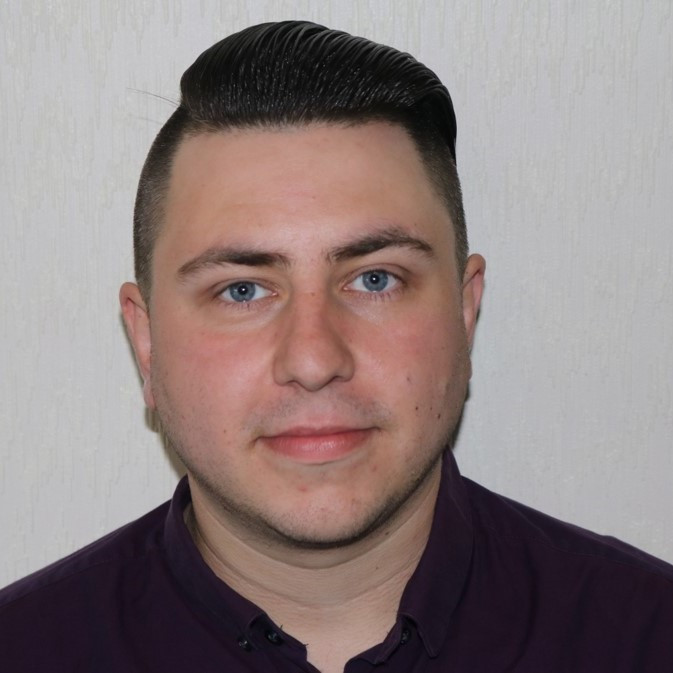