Improving Power Densities in Solid Oxide Fuel Cells with Nanoengineering
| 05-07-2021 | By Liam Critchley
As society tries to fade away from traditional fossil fuel energy systems towards greener, renewable solutions, there are a number of different options that have presented themselves (some of which are commercially feasible, others which aren’t). Fuel cells have gathered a lot of interest, especially for the next generation of vehicles, and are seen as one of the competing technologies alongside electric vehicles (but not as mature yet).
The world of fuel cells itself is a vast area, similar to that of energy generating and storage devices. One branch of fuel cells is solid oxide fuel cells (SOFCs). These types of fuel cells are known for their ability to convert chemical energy into electrical energy with high efficiency and have a large degree of flexibility about which hydrocarbon fuels can be used with them—including many common hydrocarbons such as methane and ammonia, as well as hydrogen.
There are already many commercial SOFCs on the market today that is used in real-world applications, ranging from stationary to transport applications. Over the years, there has been a lot of focus on improving the durability and long-term stability of these fuel cell systems. However, there has been a significant issue in increased costs across much of the research, so the focus has turned to nanoengineering approaches that can manipulate and tweak the properties at the molecular level—with the aim of these molecular-scale effects transforming into macro-level properties (such as increased power density and reduced stack size) without a significant cost increase. This research focuses on the cathode materials within the electrochemical cell, as these materials are easy targets for nanoengineering approaches.
Challenges of Nanoengineering Methods to Date
As a general field, Nanoengineering is the creation, manipulation, and functionalisation of materials at the nanoscale—be it on the surface of the material or within the matrix of the material. It is used to improve the properties of many materials and electronic devices, and different nanoengineering approaches can also improve SOFCs. However, many nanoengineering approaches tend to be specific to a certain property or parameter, so there is often a trade-off between what properties you want and what you can achieve. This is why many different nanoengineering methods are trialled, to see which one is the best fit for a particular material and/or device.
There have already been a number of methods that have been trialled for SOFCs, but most have some inherent challenges because while they improve some areas of the fuel cell, others get left behind (and in some cases, these can be important properties/features of the system). For example, pulsed laser deposition has been used to create cathode materials with low area-specific resistance (ASR) and high oxygen exchange properties. Additionally, non-vacuum-based sol-gel methods have been used to create lanthanum-based cathodes with very low ASR values.
However, while these approaches show some good initial properties, the focus to date on these nanoengineering cathodes has only been on the initial performance of the cathode and not the long-term stability and performance. A lot of these types of approaches have focused on producing thin-film cathodes made of only one material. So, the stability over more traditionally bulkier and porous cathodes will undoubtedly be questioned, and a lot of work on the long-term effects of these cathodes will need to be done before they are ready for real-world applications where safety and stability are some of the most important features.
Nanoengineering Hybrid Cathodes
To overcome the challenges of many of the pure thin-film electrodes, a number of nanoengineering approaches have been developed where the cathode is composed of a thin-film nanocomposite—using lanthanum materials with perovskite oxides and a good nanoscale ionic conductor material (e.g., nanoscale doped ceria).
These types of cathodes have a high cathode/electrolyte interfacial area density and oxide ion transfer that reduces the resistance throughout the system, leading to more energy-efficient transfer mechanisms. However, while they are more promising, there are still some challenges to overcome. The first is that there are not many fabrication methods that can integrate all the different elements of the composite effectively (especially the ceria materials). Secondly, on the property side, because they are still thin-film cathodes, they can lose some of their nanostructured features at high temperatures, which results in poor surface exchange properties and poor performance in the long term.
Fig 5. In the paper shows the structure of the fuel cell.
A New Nanoengineering Approach
A new approach from researchers has emerged that builds on the nanocomposite side of nanoengineering approaches. Unlike other nanocomposite efforts to date, the researchers were able to use pulsed laser deposition to create the thin-film nanocomposite, giving them a much higher degree of control over the different constituents that could be added into the nanocomposite. Other efforts using pulsed laser deposition have focused on single-material cathodes, not nanocomposites.
The team used the laser deposition method to create self-assembled layers of lanthanum strontium cobalt ferrite (LSCF) and gadolinium-doped ceria to form a densely packed nanoporous thin film (much like the bulkier porous cathodes). The distribution of these layers in the nanocomposite was found to create a highly efficient transition layer. This was found to be because of the high interfacial density and good adhesion between the layers, and the LSCF film layer providing a high surface area for the oxygen reduction reaction to take place—a mechanism that underpins many fuel cells technologies.
The resulting layered composite had high stability because of the strong adhesion between the layers and the interfacial stability. Moreover, the cathodes were found to be stable at high temperatures because the porous network and high surface area could overcome the grain coarsening effect that contributes to many of these cathodes’ high-temperature instabilities. Finally, the integration of the cathodes into conventional anode supported cells (using a thin nickel yttria-stabilized zirconia electrolyte) facilitated a reduced ohmic resistance across the cell.
In terms of specific numbers, these nanoengineered cells showed high current densities, where at 0.7 V, current densities of 2.2 and 4.7 A/cm2 were achieved at 650 °C and 700 °C, respectively, showcasing both the current density potential and thermal stability of the cell. The research here shows that tuning the material properties at the fundamental level via nanoengineering approaches offers a lower-cost route for improving the electrochemical performance of SOFCs. SOFCs are already used today, but if we are to see their use diversify into more applications, then nanoengineering approaches offer a way of increasing the power output to meet the demands of energy-intensive applications.
Reference:
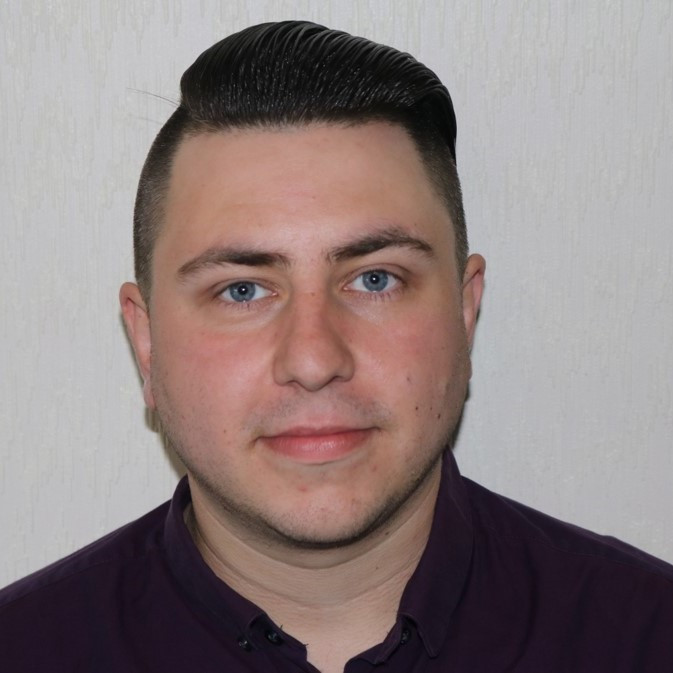