A Non-Optical Readout for Quantum Sensors
| 17-03-2021 | By Liam Critchley
Sensors have been around for a long time and are the backbone of modern detection and monitoring mechanisms—in almost every aspect of society from healthcare to weather detection. Sensors are continually getting better and advanced materials (particularly nanomaterials) have been one of the biggest class materials in recent years to revolutionise sensors.
Now, we’re looking at the next generation of sensors, known as quantum sensors. While many nanomaterials exhibit quantum effects that can contribute to quantum sensors, quantum effects can also be induced in other materials (e.g., via defects), so quantum sensors (and quantum sensing in general) are their own stand-alone field—and it is a field that is gathering a lot of interest and investment.
There’s been a lot of talk about quantum technology in the next generation of electronics. While a lot of talk centres around computers and cryptography, quantum sensing is bringing up the rear. A quantum sensor requires materials that can exhibit quantum effects. These tend to solid-state materials and nanomaterials as these materials can possess discrete quantum wells, independent of each other. At the same time, individual energy levels can be entangled and connected via electrotherapy n tunnelling.
The individual quantum states of these materials make the sensing particles much more responsive and sensitive to the local environment. The ability for quantum states to sense changes in the local environment and entangle with the rest of the material enables the measurement sensitivity to be elevated beyond what is possible with classical sensing technologies. So, while they are still in their infancy, and will need to be offered at an attractive price point to be used on a widespread commercial basis, there is a lot of potential for quantum sensors in the modern-day world (especially in high-tech applications).
Poor Readout Issues in Quantum Sensors
While there is a lot of potential for quantum sensors, one of the issues holding them back has been the readout. Like many modern-day sensors, the choice of readout in quantum sensors is often optical in nature. However, for quantum sensors, this had led to readout issues and inaccuracies. Because quantum sensors are often made of solid-state materials, the atomic lattice defects (that exhibit specific electron spins) are usually involved in the optical readout and the information's temporary storage.
Many of the solid-state defects are optically active. From sensing to the measurable and detectable output (i.e., the readout), either optical excitation or fluorescence mechanisms are used. However, the detection of these light wavelengths tends to destroy the spin defects' quantum information. This is due to the collection of photons from the emission being imperfect, so a lot of the quantum information being transferred (in the form of photons) tends to get lost.
So, in real terms, this means that a lot less information reaches the readout than was collected by the sensor at the initial point of sensing. In terms of efficiencies and sensitivities, because all the information is not collected, the sensitivities of many quantum sensors fall short of classical sensors, despite their being the potential for a much greater degree of sensitivity.
Non-Optical Readouts Using Solid-State Spin Defects
Data and information destruction issues are not ideal for monitoring and sensing the core function of the device. There has been a drive to create readouts for quantum sensors that don’t rely on transmitting the data optically. These are non-optical readouts. There has been a range of readouts already created, but most of these have focused on single (or few) spins, so many of them have not been as suitable for very high-sensitivity measurements.
Given, that the applications of quantum sensing are going to initially lie in high-tech applications (communications, medical imaging, microscopy etc.), the high theoretical sensitivities will need to be reached before we even think about commercial adoption—because it is at this point when they offer a better value proposition over classical sensors. One of the latest research pieces in the area has involved developing a non-optical readout for solid-state spin-ensemble sensors using a dielectric resonator cavity.
This cavity-based readout exploits the nitrogen-vacancy centres in diamond and leverages a strong collective coupling between a dielectric resonator cavity and a spin ensemble at room temperature. The researchers have dubbed the technique a microwave (MW) cavity readout. The readout operates by detecting changes in an applied microwave field based around the interactions between the spin ensemble and the cavity.
By tuning the microwave radiation frequency to near the resonance frequency of the spin defects, it causes absorptive and dispersive interactions to occur. These interactions encode the spin resonance in the amplitude and phase of the transmitted or reflected MWs. So, the frequency tuning enables the quantum data to be stored in the defect and transmitted, where the interactions are amplified by the cavity and then detected. Reading the spin resonance from the microwave transmission allows the data to be read in the output.
The result of this approach was a quantum data transfer and readout system that had no substantial overhead times, a narrowing of resonance features, as well as offering a way of overcoming some of the noise limitations that you find in fluorescence and optical-based readouts. All these features come together to create a readout where none of the quantum information is lost, removing one of the key issues of many quantum sensors.
There’s still a lot of work to be done in this area, but exploiting the nitrogen vacancy centres in diamond is utilised more and more for a range of technologies. So it is more viable than other options. So, while quantum sensing may take a while to manifest commercially, a lot of work is being done to improve the field's prospects. As different aspects come together, they could be realised a lot sooner than people think (which can be said for all quantum technologies given the ground that is being made in the field already).
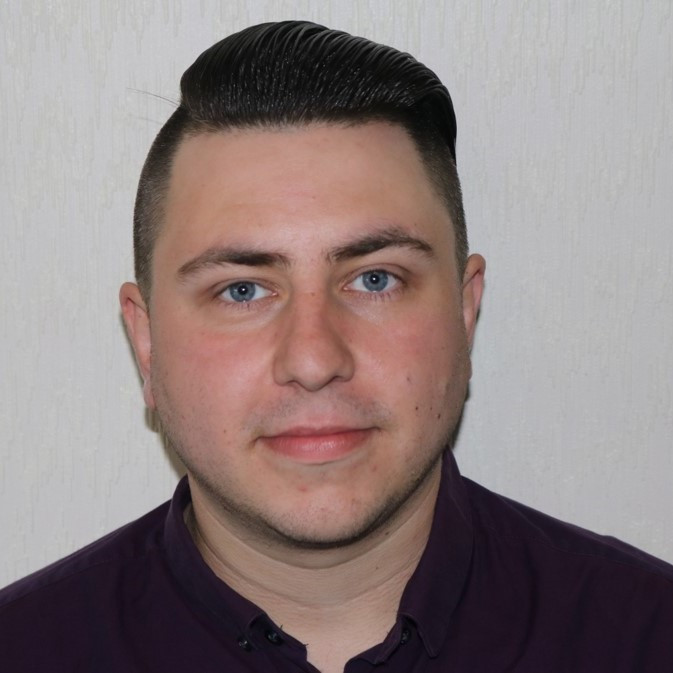