Key Considerations for Power Inverter Design in Solar and Wind Applications
| 02-09-2015 | By Microchip
The efficiency of power inverters is one of the most critical aspects of the design and a key feature for solar and wind applications. By Prasun Kulshrestha and Miguel Mendoza, Microchip Technology.
The power inverter is a widespread application for operating AC loads from a DC-operated battery system, mainly during a power failure. The power inverter must give a pure sine wave output so that the output does not damage any appliance that runs on it. The pure sine wave inverter has various applications because of its key advantages, which include operation with very low harmonic distortion and clean power such as utility-supplied electricity, reduction in audible and electrical noise in fans and cooler running of inductive loads like microwaves and motors.
The DC could be from a battery source of 12 / 24 VDC. Multiple power conversion approaches can be implemented to convert this DC from a 12VDC or 24 VDC battery to an AC voltage of 110VAC or 230 VAC.
Figure 1. Basic block Diagram of a Power Inverter
Figure 1 illustrates the basic function of a power inverter, wherein if an input AC power source is available, the AC loads would use the bypass path. Also, the battery gets charged. However, if the input AC source is unavailable (during a power failure, for example), the battery DC power could be converted into usable AC power for the electrical loads connected at the output of the power inverter system. Usually, the change over time should be equal to or greater than 10 msec so that the output loads remain unaffected by the power cut.
A power inverter's typical application is to convert battery voltage into conventional household AC voltage allowing the user to use electronic devices when AC power is not available. There are basically three kinds of inverters. The first type of inverters made, which are now obsolete, produced a square wave signal at the output. An improved type of inverter was called the modified square wave inverter, also known as the modified sine wave inverter. It produced square waves with some dead spots between positive and negative half-cycles at the output.
Pure sine wave inverters provide the cleanest utility supply. Current inverter development is going through a shift from traditional modified sine wave inverters to pure sine wave inverters because of the benefits that they offer. This is explained in Figure 2.
Figure 2. Types of Inverter Output
Figure 3 shows a pure sine wave inverter block diagram where MOSFET drivers and power devices help the design to become more efficient and compact. The approach outlined here uses two MOSFET drivers to make a full bridge topology that enables highly efficient power inverters.
System Design
First, a DC-DC power module that has an inbuilt inductor must be selected. In this example, we are using a 26V, 6A DC-DC power module that has a built-in inductor. The module incorporates a DC-DC controller, power MOSFETs, bootstrap diode, bootstrap capacitor and an inductor, all in a single package. This high level of integration simplifies design and layout for designers. Additionally, this part delivers 93% percent efficiency for 12 V and 24 V input at light load.
Figure 3. Block Diagram of Pure Sine Wave Inverter Using a Half Bridge MOSFET Driver
An additional feature of this power module is that it has short circuit protection with hiccup mode. Hiccup mode and short circuit protection help the microcontroller remain safe even during a short circuit in the battery/input. The value of the current limit protection can even be programmed to avoid error hiccup mode and can be calculated as shown in Figure 4.
Figure 4: Calculations to determine current limit protection
The power module uses fewer external components and provides a power inverter board with the smallest DC-DC power supply. Figure 5 shows the schematic of the DC-DC converter, which takes 12 VDC or 24 VDC from the battery and provides output in the form of 3.3V to the microcontroller, although the microcontroller would require only 150 to 200mA.
Figure 5. Schematic of MIC45205 power module with battery input and output going to the microcontroller
The gate driver is a power amplifier that accepts a low-power input from a controller IC and produces the appropriate high-current gate drive for a power MOSFET. The gate driver must source and sink current to establish required Vgs. The considerations for the selection of a MOSFET driver for use in a power inverter application include the following:
- Quiescent current (i.e. when LI=HI=0V) should be low, for example, 10µA, which makes battery-operated systems more efficient.
- The peak current drive capability should be targeted around 1 A to address the requirements of a power inverter for 250VA – 800VA.
- Can the half-bridge MOSFET be safely used for 12 V / 24 V battery operations? Does it have an internal bootstrap diode that allows for the use of the device for designs up to 5KW outputs?
- Can it be directly interfaced with a microcontroller?
- Does it have programmable over-voltage protection, which can be used for charge complete detection or for driver shutdown feature in case of a fault condition?
- Does it reduce the number of components with on-chip bootstrap?
- Does it offer adaptive dead time and shoot-through protection?
The power inverter's most important requirement is that two MOSFETs in a series should turn ON while the other two should remain completely OFF at any given time. If both MOSFETs pair on the side of the half-bridge while it is simultaneously ON, then VIN will short to ground. The high current from the shorted VIN supply will then "shoot through" the MOSFETs into ground. Excessive shoot-through causes higher power dissipation in the MOSFETS, voltage spikes and ringing. To avoid this, inverter designers usually use software delay to avoid the turn-on of those pair of MOSFETs simultaneously. The delay has to be selected cautiously and written into a software program, making it complex. With the right half bridge MOSFET, shoot-through can be avoided without making the system complex.
Figure 6. Dead time between gate drive output transitions.
Figure 6 shows the dead time (<20nSec) between the gate drive output transitions as the low side driver transitions from on to off while the high side driver transitions from off to on.
What are the ideal features of MOSFET drivers for a power inverter?
- Built-in active shoot-through feature simplifies the design and increases reliability
- 8-Pin SOIC packages that offer ease of use
- Programmable gate drive, which allows inverter designers flexibility in driving the MOSFETs
- The heart of the power inverter is a MOSFET driver where switching noise and shoot-through are unacceptable
Advancement of Power Inverters to Solar & Wind Power Inverters
The same power inverter design and features can be used for solar or wind power inverters, and the only difference is the input that would charge the battery. In solar inverters, sunlight is used by PV cells and is further converted to DC to charge the 12 V battery. Similarly, in the case of a wind inverter, the wind is converted to get the relative DC voltage to charge the battery. The remaining inverter stage is more or less similar to the one we discussed above for pure sine wave. The overall efficiency in solar and wind applications is the most desired requirement.
Figure 7. The functionality of solar and wind inverters
What are the distinctive requirements of solar and wind power conversion?
- System cost down: It is always important to optimise the price per output power on a system level
- System efficiency: Efficiency is most important in power conversion
- Reliability: 5+years lifetime for string inverters and 25 years for microinverters
Using the right parts for this application makes it more cost-effective. Increasing switching frequency enables cost reduction on magnetic, housing, cooling, etc. The right power module and MOSFET driver help inverter designers to achieve more efficiency.
Solar inverters may be classified into three broad types.
- Stand-alone inverters are used in isolated systems where the inverter draws its DC energy from batteries charged by photovoltaic arrays. Many stand-alone inverters also incorporate integral battery chargers to replenish the battery from an AC source when available. Usually, these do not interface in any way with the utility grid and, as such, are not required to have anti-islanding protection.
- Grid-tie inverters, which match phase with a utility-supplied sine wave. Grid-tie inverters are designed to shut down automatically upon loss of utility supply for safety reasons. They do not provide backup power during utility outages.
- Battery backup inverters are special inverters designed to draw energy from a battery, manage the battery charge via an onboard charger, and export excess energy to the utility grid. These inverters are capable of supplying AC energy to selected loads during a utility outage and are required to have anti-islanding protection.
Conclusion
With the correct solutions for power inversion, designers can understand how the right power management devices are beneficial. Having features like shoot-through protection and adaptive dead time allows designers to use hardware-defined delay. The system becomes more robust as the shoot-through can be avoided with the hardware itself.
The most important aspect of power inverters, solar, and wind inverters is the need for the most efficient system. The power inverter becomes more efficient with a power module capable of delivering 93% percent of the rated load and the lowest quiescent current.
Since most of the advancements in technology for power inverters are towards pure sine wave inverters, it is essential to choose the MOSFET driver with the most appropriate fall and rise time, inbuilt shoot-through feature and current driving capability.
Authors:
Prasun Kulshrestha - Prasun Kulshrestha has over 9 years of experience in the semiconductor industry. After spending 4 years in research and design development of SMPS-based power supplies from 0.5 Watts to 410 Watts, he worked on 800VA sine wave power inverters for battery-operated power backup applications. For the past 4 years, Prasun has worked for Micrel as Senior Application Engineer based in Delhi, India, in the company's power inverter business, focusing mainly on MOSFET drivers and power inverter design. He has core expertise in power and analogue electronics and has designed several SMPS power supplies for various applications such as TVs, set-top boxes, battery chargers, LEDs and DVD players, including non-isolated and isolated designs. Prasun holds a Bachelor's Degree in Engineering in Electronics and Communications from the University of Nagpur, India.
Miguel Mendoza - Marketing Product Manager, Linear Power Solutions - Miguel Mendoza has 14 years of high-tech experience in the semiconductor industry. He is currently based in Micrel headquarters in San Jose, where he is responsible for Automotive and Industrial Product Marketing for power management, MOSFET drives and LED driver solutions. Prior to coming to Micrel, Mendoza worked for several high-tech companies in various roles, including as product marketing manager at Atmel and Maxim Integrated Products, where he gained more than a decade of product marketing experience in interface products, LED backlighting for television and Solid State lighting. Mendoza graduated with a bachelor's degree in Electrical Engineering from California Polytechnic University San Luis Obispo.
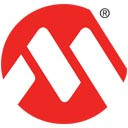